Translate this page into:
Anatomic reconstruction techniques for posterolateral corner injuries: Current concepts in management and rehabilitation
*Corresponding author: Robert F. LaPrade, Department of Orthopedic Surgery, Twin Cities Orthopedics, Edina, United States. laprademdphd@gmail.com
-
Received: ,
Accepted: ,
How to cite this article: Nassar JE, Sleem B, Tollefson LV, LaPrade RF. Anatomic reconstruction techniques for posterolateral corner injuries: Current concepts in management and rehabilitation. J Arthrosc Surg Sports Med. doi: 10.25259/JASSM_63_2024
Abstract
Background and Aims
Posterolateral corner (PLC) injuries are complex knee injuries that compromise stability due to damage to critical stabilizing structures, requiring accurate diagnosis, effective surgical techniques, and structured rehabilitation for optimal outcomes. This review explores the anatomy, biomechanics, and diagnostic strategies for PLC injuries, emphasizing on LaPrade’s anatomical reconstruction technique and its role in restoring stability and function.
Materials and Methods
A comprehensive literature search was conducted across PubMed, Scopus, Embase and Web of Science for studies focusing on PLC anatomy, diagnostics, physical examination techniques, imaging, treatment approaches, and postoperative rehabilitation.
Results
The review highlights the roles of the fibular collateral ligament, popliteus tendon, and popliteofibular ligament in resisting varus forces and controlling knee rotation. Diagnostic approaches, including physical examinations, radiographs, and magnetic resonance imaging, are discussed to identify PLC injuries effectively. Surgical management focuses on anatomical reconstruction techniques, particularly LaPrade’s technique, to restore native biomechanical functions. Post-operative rehabilitation protocols are emphasized, with a structured approach to restoring stability, minimizing complications, and improving functional outcomes.
Conclusion
Combining anatomic reconstruction with a rigorous rehabilitation protocol has demonstrated favorable subjective and objective outcomes, providing an effective framework for managing PLC injuries and achieving long-term functional recovery.
Keywords
Anatomic reconstruction
Fibular collateral ligament
Popliteofibular ligament
Popliteus tendon
Posterolateral corner injury
INTRODUCTION
The posterolateral corner (PLC) of the knee, historically termed the “dark side of the knee,” poses a challenge to treat due to its complex structures, biomechanics, and treatment approaches.[1] Recent advancements in anatomic knowledge and biomechanically validated reconstruction techniques have improved the management of PLC injuries, which are often associated with anterior cruciate ligament (ACL) or posterior cruciate ligament (PCL) tears, with only around 13-28% occurring in isolation.[1-4] If not adequately addressed, PLC injuries can alter knee biomechanics, compromise cruciate ligament reconstructions, and accelerate joint degeneration.[5-7]
These injuries are increasingly recognized due to improvements in diagnostics and rising sports-related trauma and motor vehicle accidents.[8,9] High-energy traumatic events, such as football, soccer, skiing, and vehicular collisions, are common causes, although non-contact hyperextension or varus injuries can also damage the PLC.[8,10] Complete grade III injuries typically involve disruption of the fibular collateral ligament (FCL), popliteus tendon (PLT), and popliteofibular ligament (PFL).[1,11]
Up to 70% of PLC injuries may be initially missed, necessitating a high index of suspicion and detailed evaluation through physical examination, radiography, and magnetic resonance imaging (MRI).[4,12] Non-operative treatments for grade III injuries have shown poor outcomes, making reconstruction essential for restoring varus and rotational stability, especially in conjunction with cruciate ligament reconstructions.[13-15] Anatomic reconstruction is favored for its ability to restore natural knee biomechanics more accurately than repairs.[16-19]
This narrative review aims to summarize the latest concepts on PLC injuries, including the anatomy, biomechanics, diagnostics, treatment strategies, and outcomes, serving as a practical guide for effective management.
MATERIALS AND METHODS
A comprehensive literature search was conducted across PubMed, Scopus, Embase, and Web of Science from inception through December 10, 2024. The search terms included “posterolateral corner injuries,” “PLC injuries,” “PLC repair,” and “PLC reconstruction.” Inclusion criteria encompassed studies that focused on PLC anatomy, diagnostics, physical examination techniques, imaging, treatment approaches, or post-operative rehabilitation. Exclusion criteria included case reports, conference abstracts, non-English studies, and articles without full-text availability. The quality of the included studies was assessed based on methodological rigor, relevance to the topic, and strength of evidence. Studies with significant limitations or lacking sufficient data were excluded from the study.
RESULTS
Anatomy and assessment of PLC injuries
The PLC anatomy is crucial for knee stability, counteracting varus forces, and controlling rotational movements.[1] The lateral knee’s complex bony structure, including the convex lateral femoral condyle (LFC) and lateral tibial plateau (LTP), creates inherent instability, complicating the healing of severe grade III injuries.[14,20] The primary stabilizers, including the FCL, PLT, and PFL, each uniquely contribute to knee stability [Figure 1].[21]
![Photograph (a) and illustration (b) showcasing the isolated structures of the fibular collateral ligament, popliteus tendon, popliteofibular ligament, and lateral gastrocnemius tendon from a lateral perspective of the right knee (Reproduced with permission from LaPrade et al.).[21]](/content/115/2025/0/1/img/JASSM-63-2024-g001.png)
- Photograph (a) and illustration (b) showcasing the isolated structures of the fibular collateral ligament, popliteus tendon, popliteofibular ligament, and lateral gastrocnemius tendon from a lateral perspective of the right knee (Reproduced with permission from LaPrade et al.).[21]
The FCL attaches to a shallow depression on the femur, 1.4 mm proximal and 3.1 mm posterior to the lateral epicondyle, extending to the lateral fibular head, 8 mm posterior to its anterior aspect.[4,21] Measuring about 70 mm in length, the FCL is the primary static restraint to varus movement and resists external knee rotation, particularly during extension. It also provides secondary stability for internal rotation and anterior tibial translation.[4,19,22,23] Varus gapping >2.2-2.7 mm compared to the contralateral knee indicates an isolated FCL tear.[4,24]
The PLT originates from the anterior fifth of the popliteal sulcus and inserts broadly on the posteromedial tibial cortex, spanning about 54.9 mm.[1] Positioned deep to the FCL, it primarily restrains external tibial rotation relative to the femur. The PLT also anchors to the lateral meniscus at three popliteomeniscal fascicles, enhancing knee stability.[22,25,26] Its femoral insertion, the most anterior PLC attachment, is located 18.5 ± 1.5 mm anterior to the FCL attachment when the knee is at 70° [Figure 2].[4,22]
![The lateral view of the right knee highlighting the attachment points of the fibular collateral ligament (FCL) on the femur and fibula, as well as the popliteus tendon (PLT) within the femoral popliteus sulcus. The average distance between the femoral attachment sites is also indicated. (Reproduced with permission from LaPrade et al.).[21]](/content/115/2025/0/1/img/JASSM-63-2024-g002.png)
- The lateral view of the right knee highlighting the attachment points of the fibular collateral ligament (FCL) on the femur and fibula, as well as the popliteus tendon (PLT) within the femoral popliteus sulcus. The average distance between the femoral attachment sites is also indicated. (Reproduced with permission from LaPrade et al.).[21]
The PFL originates at the popliteus musculotendinous junction and attaches distally to the posteromedial aspect of the fibular styloid process with two divisions: One near the styloid tip and the other slightly distal,[20,22] forming an overall 83° angle with the PLT during surgery.[8,21] The PFL serves as a key static restraint for external knee rotation and a secondary stabilizer against varus rotation. Its role in stability becomes evident when injured with other PLC structures, as reconstructing the PFL along with the FCL and PLT significantly improves knee stability in grade III PLC injuries.[17,27]
Several secondary structures enhance posterolateral knee stability by reinforcing the restraints provided by the FCL, PLT, and PFL.[1] The biceps femoris complex serves as a key dynamic stabilizer. Its long head divides into direct and anterior arms, inserting near the fibular styloid and the FCL attachment, respectively, forming a supportive connection to the FCL.[22,26,28] The short head splits into components that attach to the lateral tibia and the posterolateral joint capsule, further stabilizing the knee.
The distal iliotibial band (ITB) contains deep fibers, known as Kaplan fibers, which play a critical role in stabilizing the distal femur and lateral knee structures.[22,26,28] These fibers are categorized into two bundles: proximal and distal Kaplan fibers.[29] The proximal fibers anchor to the proximal ridge of the distal femoral diaphysis, approximately 53.6 mm above the lateral epicondyle, while the distal fibers attach to the distal ridge on the supracondylar flare, located 31.4 mm proximal to the lateral epicondyle [Figure 3].[29] The ITB contributes significantly to posterolateral stability not only through its distal attachment but also through its dynamic interactions with other structures. The distal iliopatellar band connects the ITB to the patella, while the deep capsuloosseous layer integrates with the lateral gastrocnemius muscle, biceps femoris tendon and tibia.[1,22,26] These connections create a robust network that reinforces the knee’s lateral stability, particularly during rotational or valgus stress. The anterolateral ligament (ALL) contributes to rotational stability, particularly in controlling internal tibial rotation, and serves as a secondary restraint to anterior tibial translation in ACL-deficient knees.[30] Positioned between the LFC and proximal tibia, it plays a key stabilizing role.[30] The lateral joint capsule, with its meniscotibial ligament connecting the lateral meniscus to the posterior tibia, adds further support. Finally, the fabellofibular ligament, linking the fabella to the fibular head, supports external rotation and hyperextension while resisting varus forces by anchoring to the lateral gastrocnemius tendon and joint capsule.[22,26]
![A lateral dissection of a right femur illustrating the orientation, origin, and insertion points of the proximal and distal Kaplan fibers. FCL: Fibular collateral ligament, GT: Lateral gastrocnemius tendon, ITB: Iliotibial band, PLT: Popliteus tendon, DKF: Distal Kaplan fibers, PKF: Proximal Kaplan fibers (Reproduced with permission from Godin et al.).[29]](/content/115/2025/0/1/img/JASSM-63-2024-g003.png)
- A lateral dissection of a right femur illustrating the orientation, origin, and insertion points of the proximal and distal Kaplan fibers. FCL: Fibular collateral ligament, GT: Lateral gastrocnemius tendon, ITB: Iliotibial band, PLT: Popliteus tendon, DKF: Distal Kaplan fibers, PKF: Proximal Kaplan fibers (Reproduced with permission from Godin et al.).[29]
The Hughston classification system
The Hughston classification subjectively assesses knee stability under varus stress with the knee fully extended, categorizing ligament injuries into three grades [Table 1].[31,32] Grade I indicates minimal tearing with no abnormal motion and a stable knee. Grade II involves partial tearing with mild-to-moderate abnormal motion, showing some instability that may require conservative or surgical treatment. Grade III represents a complete ligament tear with significant abnormal motion, typically necessitating surgery in active patients. While subjective and prone to overestimating lateral compartment gapping compared to anatomic studies, this classification remains a valuable tool for clinicians in assessing and communicating injury severity and guiding treatment.[31,32]
Classification | Varus or rotational instability | PLC injury |
---|---|---|
Grade I | 0-5 mm or 0-5° | Minimal tearing with no abnormal motion |
Grade II | 5-10 mm or 6-10° | Partial tearing with mild-to-moderate abnormal motion |
Grade III | >10 mm or >10° (soft endpoint) | Complete tearing with marked abnormal motion |
PLC: Posterolateral corner
History and physical examination
Grade III PLC injuries often occur alongside other ligament injuries rather than in isolation.[4,33] Patients typically recall a specific traumatic event, such as hyperextension with twisting, a direct blow to the anteromedial tibia in extension, landing on an outstretched leg, or a high-energy impact.[1,34] Common symptoms include localized pain, swelling, tenderness on the lateral knee, instability near full extension, difficulty walking on uneven surfaces or navigating stairs, sometimes presenting as a varus thrust gait.[35] Injuries may also affect the common peroneal nerve, causing paresthesias or a foot drop.[33,36] A thorough physical examination is crucial, utilizing tests such as varus stress, dial, posterolateral drawer, reverse pivot shift, and external rotation recurvatum, performed bilaterally for comparison.[1,4]
The varus stress test evaluates lateral compartment gapping compared to the contralateral knee in full extension and at 20-30° of flexion.[4] Gapping at 20-30° suggests FCL and potentially other PLC injuries while gapping in full extension indicates possible cruciate ligament involvement.[4] The dial test measures rotational stability by comparing external rotation at 30° and 90° of knee flexion. Increased rotation at 30° with posteromedial subluxation suggests PLC injury, while continued rotation at 90° indicates combined PCL and PLC involvement.[4,34,37] A positive dial test with anteromedial subluxation may also indicate a grade III MCL tear.[38]
The reverse pivot shift test assesses rotational stability by applying valgus force and external rotation to a flexed knee during gradual extension. A reduction at 35-40° of flexion indicates PLC injury as the ITB transitions from a flexor to an extensor.[1,4,33,34] However, a study by Cooper found a positive sign in 35% of normal knees, highlighting the importance of contralateral comparison.[39] The external rotation recurvatum test involves lifting the leg by the great toe in a supine position and comparing heel height to the unaffected side. Increased heel height suggests a PLC injury and possible ACL tear [Figure 4].[34,40-42]
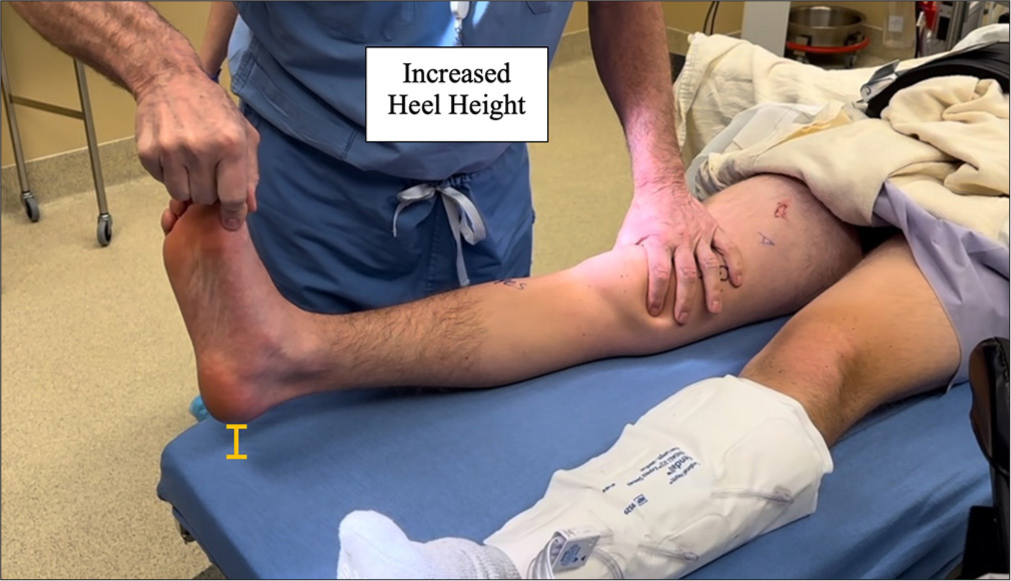
- The external rotation recurvatum test evaluates the increased heel height commonly seen in patients with posterolateral corner (PLC) injuries. Initially used to gauge the severity of PLC tears, it is now recognized as a key indicator of combined injuries involving the anterior cruciate ligament (ACL) and PLC. A heel height difference exceeding 2.5 cm between sides suggests a combined ACL and fibular collateral ligament tear. During the test, the distal thigh is stabilized with one hand while the foot is lifted using the great toe with the other hand. In cases of combined PLC and ACL injuries, anterior tibial subluxation and external rotation lead to a noticeable increase in heel height.
Imaging
When a PLC injury is suspected after a thorough history and physical examination, imaging is essential for confirmation.[4] Initial assessment typically includes standard anteroposterior, lateral, and flexed knee patellofemoral views to screen for fractures or avulsions, though these are often normal in acute PLC injuries.[1] For chronic cases, a standing long-leg anteroposterior alignment radiograph is recommended to detect varus malalignment, which should be corrected with a bi-planar osteotomy before or during PLC reconstruction.[4] Varus stress radiographs provide a reliable method to evaluate PLC and FCL integrity, particularly in grade III FCL tears. Taken bilaterally at 20° of knee flexion, they measure lateral compartment gapping as the shortest distance between the LFC and LTP.[4] A side-to-side difference (SSD) in varus gapping over 4mm indicates a complete PLC tear, while an SSD of 2.2-4 mm suggests an isolated FCL tear [Figure 5].[24,43] Stress radiographs, though challenging in acute settings, are more accurate than MRI for diagnosing chronic grade III FCL injuries.[44,45] MRI, with a sensitivity of 58% to 100% for PLC injuries, provides valuable insights into isolated FCL injuries and comprehensive PLC damage, especially in the acute phase, where its sensitivity is higher.[46,47] Acute PLC injuries on MRI often show anteromedial bone bruising.[12] In a study by Geeslin and LaPrade, 55% of acute PLC injuries displayed bruising on the anteromedial femoral condyle, and nearly 30% showed posteromedial tibial plateau bruising, frequently associated with ACL injuries.[2] Anteromedial bone bruising or medial tibial plateau fractures on MRI strongly suggest a PLC injury unless proven otherwise [Figure 6].[1]
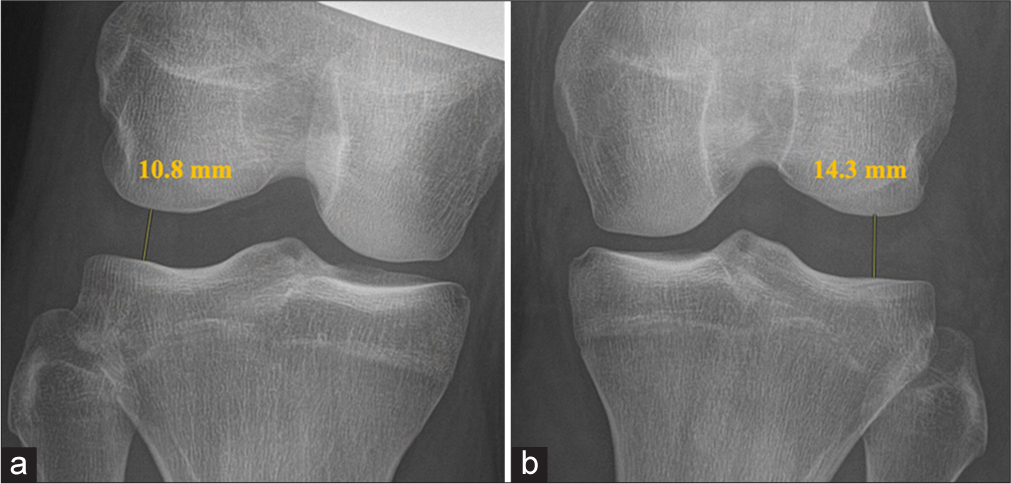
- (a and b) Bilateral varus stress radiographs of the posterolateral corner (PLC). The left knee (b) exhibits 3.5 mm of additional lateral compartment separation. A side-to-side difference in varus gapping of more than 2.2 mm typically points to an isolated fibular collateral ligament tear, whereas a gap exceeding 4 mm suggests a complete rupture of the PLC.
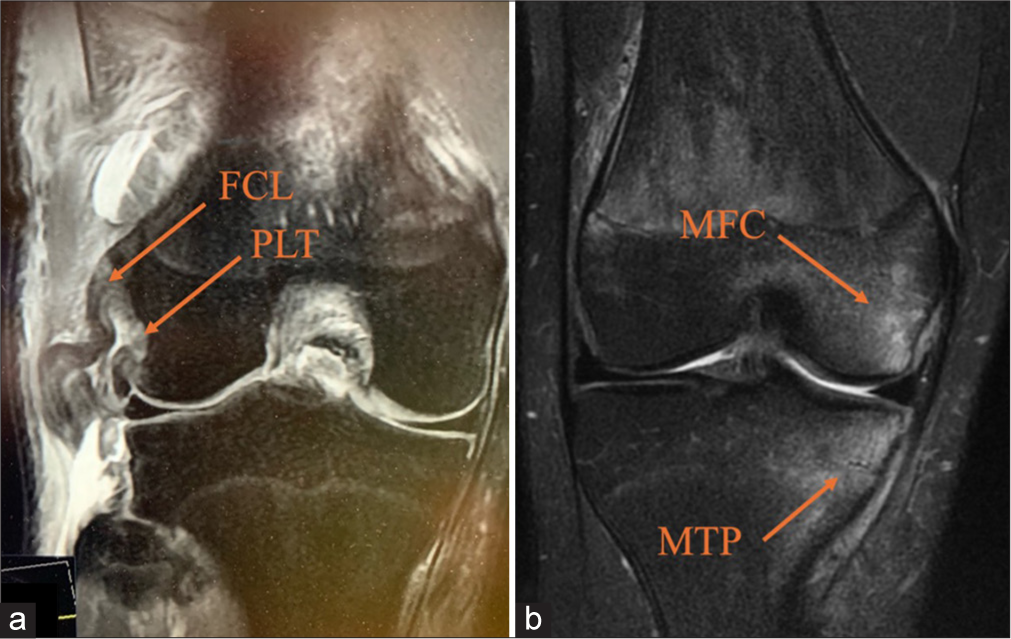
- (a) A coronal magnetic resonance imaging (MRI) of the right knee displays fibular collateral ligament (FCL) attenuation and a popliteus tendon (PLT) avulsion, marked by arrows. (b) Acute posterolateral corner injuries frequently present with anteromedial bone bruising, observed in up to 55% of cases. This coronal MRI of the right knee shows increased signal intensity in the medial femoral condyle (MFC) and medial tibial plateau (MTP).
Treatment
The treatment of PLC injuries varies based on the injury grade and the extent of the associated ligament damage. Non-operative management is typically reserved for grade I and II injuries, while surgical options are recommended for more severe injuries or when concurrent ligament damage is present.[48,49]
Non-operative treatment is indicated for injuries that only involve partial midsubstance tears without avulsions.
In fact, studies have shown good results for these lower-grade injuries when managed conservatively, with reports of minimal radiographic changes at an eight-year follow-up time.[50-53] This approach includes a period of knee immobilization that is followed by a controlled rehabilitation that focuses on gradual mobilization, strengthening, and stability exercises. However, non-operative management for complete grade III injuries is generally discouraged because it often leads to poor functional outcomes, persistent instability, and increased degenerative changes.[11,15] If these injuries are left untreated, excessive strain on concurrent PCL and ACL grafts can compromise their integrity.[5,6]
Surgical intervention becomes necessary for more severe PLC injuries, especially isolated acute grade II avulsions or grade III injuries with complete disruption of PLC structures.[4] Early surgical intervention, which is ideally within 2-3 weeks from the time of injury, is especially effective for isolated avulsions of the FCL and PLT without midsubstance damage.[4] In fact, early repair is indicated in acute settings where direct reattachment of avulsed structures is feasible and may offer biomechanical advantages over reconstruction by preserving native proprioception.[54] The technique involves anatomically reattaching the avulsed structures using suture anchors or screw fixation, depending on the site of detachment.[54] For FCL bony avulsions, fixation with cannulated screws or cortical buttons has shown favorable outcomes when performed within the acute window before scar formation and ligament retraction.[54]
For severe PLC injuries, the LaPrade anatomical reconstruction technique is widely regarded for its stability to restore the knee’s native structure and function [Table 2].[1,20,55] This technique restores the FCL, PLT, and PFL with the use of an Achilles tendon allograft, which is split in length into two separate grafts. Each graft is prepared with a 9x20 mm bone plug on one end and tubularized on the other end.[17] Studies have also shown that hamstring autografts could be a viable option.[56] The procedure begins with a lateral hockey incision, followed by posterior dissection along the distal ITB and both the long and short biceps femoris muscles. In cases of long biceps tendon rupture, special care is advised as scar tissue or malalignment could obscure the potential altered course of the common peroneal nerve. A neurolysis is performed to decompress the nerve, which is gently retracted for protection. The distal FCL attachment to the lateral fibular head is identified by entering the biceps bursa between the anterior and direct arms of the long head of the biceps femoris. A traction suture can be placed in the distal FCL remnant to assess its integrity and help in localizing the femoral attachment which also assists in guiding the ITB splitting incision if some ligament continuity is present.[15] Retracting the injured biceps femoris tendon or creating a longitudinal incision through the anterior arm of the long head at approximately 1 cm proximal to the fibular head is needed to provide a clearer view of the distal FCL attachment [Figure 7].[4]
Step | Pearls | Pitfalls | Improved recommendations |
---|---|---|---|
Anatomic exposure | Begin with a posterolateral approach to clearly visualize anatomy before fluid extravasation | Scarring or an avulsed BF tendon makes the common peroneal nerve location unpredictable. Proceed slowly with neurolysis | Use intraoperative nerve monitoring to further reduce the risk of nerve damage |
Identifying fibular attachments | Incise the biceps bursa to locate the FCL attachment (28 mm distal to fibular styloid, 8 mm posterior to the anterior fibular head) | Reaming the fibular head tunnel too proximally risks fracture or blowout | Confirm fibular head positioning with fluoroscopic guidance to reduce the risk of misplacement |
Tagging ligaments | Tag any remnant FCL tissue to assist in identifying the femoral FCL origin accurately | Incorrect femoral pin placement (<18.5 mm apart for FCL and PLT pins) risks tunnel convergence | Double-check pin spacing using intraoperative calipers before proceeding with reaming |
Tibial tunnel preparation | Use an obturator inside the fibular head tunnel to guide the trajectory of the tibial PLC tunnel | A lateral PLC tibial guide pin can pierce the anterior compartment or the proximal tibiofibular joint, especially in obese patients | Preoperatively mark guide pin trajectories on fluoroscopy to minimize lateral deviation |
Tunnel exit strategy | Ensure the tibial tunnel exits 1 cm medial and 1 cm proximal to the posteromedial exit of the fibular tunnel | Incorrect trajectory during tunnel creation can result in failed graft alignment | Use a calibrated tibial guide system to maintain consistent exit points |
Soft tissue handling | Make femoral ITB incision anteriorly to simplify retraction during tunnel preparation | Posterior or inferior incisions make soft tissue retraction more challenging | Place a tensioning device to maintain a clear surgical field during ITB handling |
Neurovascular protection | Protect neurovascular structures using a large Chandler retractor with a finger behind it during drilling | Insufficient retraction risks damage to the neurovascular bundle | Reinforce retraction with an assistant holding a secondary retractor for added safety |
Locating the femoral origin | Perform a small arthrotomy to identify the PLT femoral origin; measure 18.5 mm posterior to locate the FCL origin | Difficulty in visualizing landmarks can delay surgery | Pre-mark potential insertion points on radiographs before the procedure for quicker localization |
Femoral tunnel trajectory | Aim femoral tunnels 35-40° proximal and posterior to prevent tunnel convergence with ACL tunnels | Tunnel convergence reduces graft stability and leads to reconstruction failure | Use an angled guide specific to PLC reconstructions to ensure accurate femoral tunnel trajectories |
Graft passage | Pass grafts under the ITB, ensuring the PLT is deep to the FCL graft. | Improper graft passage can compromise graft function. | Test graft tension and alignment intraoperatively to verify correct placement. |
Graft fixation | The FCL should be fixated at 20° of flexion and the PLT and PFL should be fixated at 60° of flexion. | Fixating the grafts at incorrect fixation angles can lead to loose grafts and decreased biomechanical function. | A slight varus force during fixation can help ensure grafts are properly tensioned. |
Repair of BF tendon | Repair the BF tendon in full extension post-reconstruction to avoid convergence between anchors and fibular tunnel | Improper repair can result in limited knee extension postoperatively | Anchor placement should be finalized after trial graft tensioning to confirm non-interference zones |
FCL: Fibular collateral ligament, BF: Biceps tendon, PLC: Posterolateral corner, PLT: Popliteus tendon, ITB: Iliotibial tendon, PFL: Popliteofibular ligament
![The fibular collateral ligament (FCL), popliteus tendon (PLT), and popliteofibular ligament (PFL) serve as the primary stabilizing structures of the posterolateral corner. Additional structures shown include the lateral gastrocnemius tendon (LGT), lateral meniscus (LM), and the long head of the biceps femoris tendon (BFT), which inserts on the lateral side of the fibular head (FH). Typically, the anterior arm of the BFT conceals the distal attachment of the FCL. However, in this image, the anterior arm has been removed to provide a clear view of the distal FCL attachment, which is normally situated within a bursa between the anterior and direct arms of the biceps femoris tendon (Reproduced with permission from LaPrade et al.).[1]](/content/115/2025/0/1/img/JASSM-63-2024-g007.png)
- The fibular collateral ligament (FCL), popliteus tendon (PLT), and popliteofibular ligament (PFL) serve as the primary stabilizing structures of the posterolateral corner. Additional structures shown include the lateral gastrocnemius tendon (LGT), lateral meniscus (LM), and the long head of the biceps femoris tendon (BFT), which inserts on the lateral side of the fibular head (FH). Typically, the anterior arm of the BFT conceals the distal attachment of the FCL. However, in this image, the anterior arm has been removed to provide a clear view of the distal FCL attachment, which is normally situated within a bursa between the anterior and direct arms of the biceps femoris tendon (Reproduced with permission from LaPrade et al.).[1]
Using an elevator, the soleus muscle is carefully elevated from the posteromedial fibular head to expose the PLT and PFL musculotendinous junction. A guide pin is inserted at the FCL origin, positioned 8 mm posterior to the anterior margin of the fibular head, and directed in an anterolateralto-posteromedial trajectory to exit just beneath the PFL attachment.[4,17] The fibular tunnel is reamed with a 7 mm reamer to facilitate proper graft placement.
For the transtibial tunnel, a guide pin is drilled starting from the flat surface just distal and medial to Gerdy’s tubercle, extending to the posterior popliteus musculotendinous junction. The exit point is confirmed to be approximately 1 cm medial and 1 cm proximal to the posteromedial fibular tunnel opening.[4,17] A retractor is placed to protect the neurovascular structures during this process. Subsequently, the tibial tunnel is reamed with a 9 mm reamer.
The longitudinal incision of the ITB is essential to locate the femoral FCL attachment, which lies approximately 1.4 mm proximal and 3.1 mm posterior to the lateral epicondyle.[21] After identifying the sulcus, any remaining FCL fibers at the attachment site are removed. An eyelet guide is drilled at a proximal and 35° anterior angle to avoid convergence with ACL reconstruction tunnels.[57] This trajectory reduces risks associated with tunnel interference.
Finally, the PLT attachment is identified within the popliteus sulcus and lies 18.5 mm anterior to the FCL attachment, serving as a critical anatomical reference point for reconstruction [Figure 8].[21]
![(a and b) Anatomical reconstruction of the posterolateral corner (PLC). These images showcase the completed appearance of a PLC reconstruction, designed to restore the natural structure of the fibular collateral ligament (FCL), popliteofibular ligament (PFL), and popliteus tendon (PLT). The procedure utilizes a longitudinally split Achilles tendon allograft, secured with a fibular head tunnel, a transtibial tunnel, and interference screws for fixation (Reproduced with permission from LaPrade et al.).[20]](/content/115/2025/0/1/img/JASSM-63-2024-g008.png)
- (a and b) Anatomical reconstruction of the posterolateral corner (PLC). These images showcase the completed appearance of a PLC reconstruction, designed to restore the natural structure of the fibular collateral ligament (FCL), popliteofibular ligament (PFL), and popliteus tendon (PLT). The procedure utilizes a longitudinally split Achilles tendon allograft, secured with a fibular head tunnel, a transtibial tunnel, and interference screws for fixation (Reproduced with permission from LaPrade et al.).[20]
A second eyelet guide pin is placed parallel to the first pin or instead, the PLT pin can be placed first through a small arthrotomy to locate the PLT tendon in the anterior fifth of the popliteal sulcus. Before starting to ream, one should ensure that the pins are spaced roughly 18.5 mm apart. Both pins are then over-reamed with 9 mm reamers to a depth of 25 mm [Figure 9].
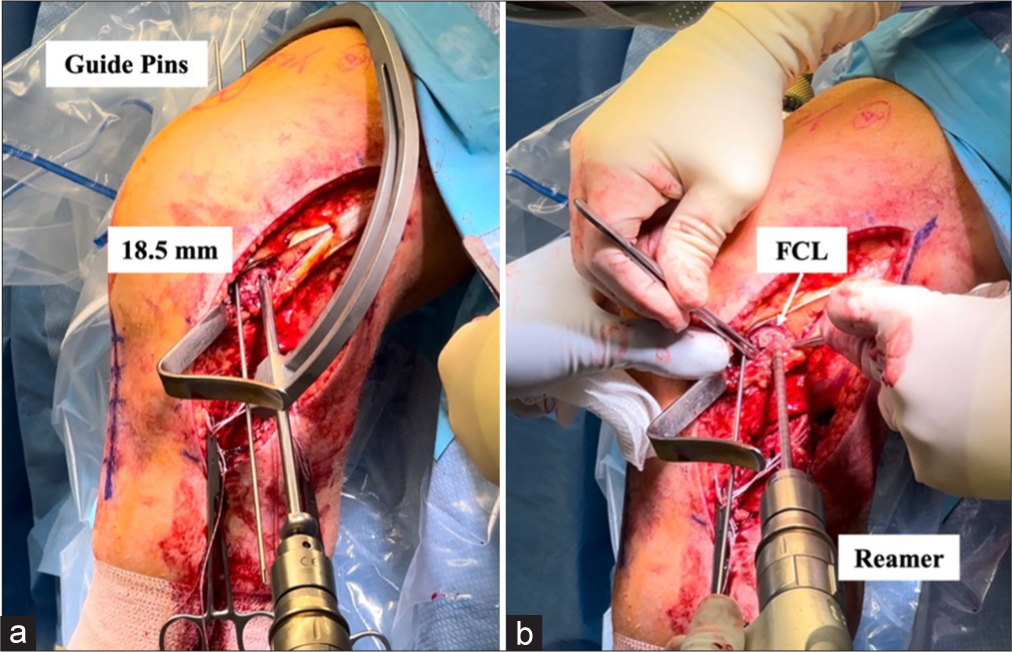
- (a and b) Creating femoral tunnels for the fibular collateral ligament (FCL) and popliteus tendon (PLT) grafts. The FCL attaches anatomically near the lateral epicondyle located approximately 18.5 mm posterior to the PLT attachment within the popliteal sulcus (a). In reconstructing the posterolateral corner (PLC), the process begins with the placement of a guide pin at the PLT’s femoral attachment. After verifying the correct spacing, a second guide pin is positioned for the FCL tunnel. Once both guide pins are accurately placed, a 9 mm reamer is used to drill femoral tunnels for the grafts, each reaching a depth of 25 mm (b).
To ensure precise graft placement, passing stitches are first inserted using eyelet guide pins, which facilitate the smooth threading of the grafts into their designated femoral tunnels. Once positioned, the bone plugs of both the PLT and FCL grafts are secured within their respective tunnels.
The PLT graft is carefully routed through the popliteal hiatus, maintaining a posterior and distal trajectory to align with the native ligament’s anatomical course. Meanwhile, the FCL graft is passed superficial to the PLT graft but remains deep to the ITB, preserving the biomechanical relationships between the reconstructed structures.
For the fibular head tunnel, the FCL graft is directed from lateral to medial and anchored securely within the tunnel using a 7 × 20 mm bioabsorbable interference screw. This critical step is performed with the knee positioned at approximately 20° of flexion, maintained in neutral rotation, and with a controlled valgus force applied to replicate the native ligament tension. These intraoperative adjustments enhance the restoration of knee stability while minimizing the risk of over-tightening the graft.
Emerging from the posteromedial aperture of the fibular tunnel, the continuation of the graft serves as the PFL graft. Alongside the PLT graft, this segment is carefully passed from posterior to anterior through the tibial tunnel. Ensuring proper alignment in the tibial tunnel is vital to preventing tunnel convergence and achieving consistent load distribution across the grafts.
To improve procedural efficiency, pre-tensioning of the grafts can be performed before final fixation to account for potential slack and optimize post-operative ligament tension. The final fixation is performed with the use of a 9 × 20 mm bioabsorbable interference screw with the knee flexed at 60° and in neutral rotation [Figure 10].[1] This precise placement and fixation method restores both the varus and the rotational stability of the knee, which allows for an effective load-bearing while reducing the risk of recurrent instability or graft stretching.[1] The LaPrade anatomical reconstruction technique has been reported to be biomechanically validated in restoring the stability of the knee and provides favorable outcomes in the management of PLC injuries.[17,27]
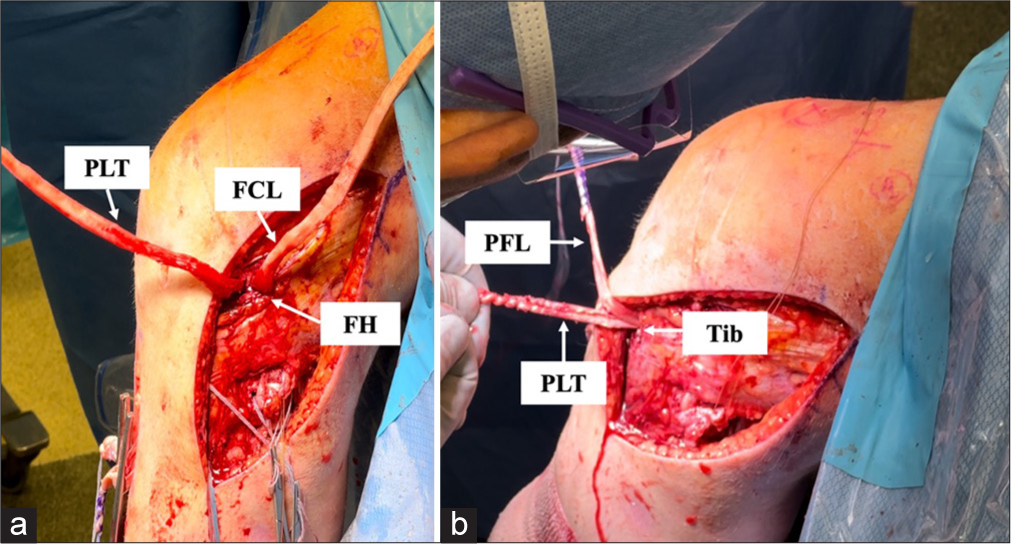
- An intraoperative image demonstrating an anatomic reconstruction of the posterolateral corner. This approach utilizes two grafts to restore the function of three key structures: (a) The fibular collateral ligament (FCL), popliteus tendon (PLT), and popliteofibular ligament (PFL). (b) The FCL graft is routed anterolaterally to posteromedially through a tunnel in the fibular head (FH) and, together with the PLT graft, is passed from posterior to anterior through a tibial tunnel. After securing the FCL graft at the fibular head, its remaining segment functions as the PFL graft, contributing to the stabilization of the proximal tibiofibular joint.
Tunnel convergence
In PLC reconstructions, it is essential to avoid tunnel convergence to maintain the integrity of the grafts and provide an effective fixation. Tunnel convergence occurs when drilled tunnels intersect within the bone which would potentially compromise the graft stability and increase the risk of fixation failure.[57] Proper planning of tunnel trajectories, especially for the FCL, PLT, and ACL, is critical to ensure structural separation and prevent complications in complex knee reconstructions. In studies by Moatshe et al., it was reported that angling the FCL and PLT tunnels 35-40° anteriorly and maintaining a neutral vertical angle (0° proximally) on the femur can help avoid convergence with the ACL tunnel [Figure 11].[57-59] When the FCL tunnel is drilled at 0° in both coronal and axial planes, the risk of convergence with the ACL tunnel reaches 100%.[57,58]
![To prevent tunnel convergence with the anterior cruciate ligament (ACL) reconstruction, the femoral tunnel for the fibular collateral ligament (FCL) should be drilled at a 35° anterior angle. When the patient is positioned supine, the surgeon lowers their hand while angling the reamer upward to achieve this trajectory. The tunnel for the popliteus tendon (PLT) is aligned parallel to the FCL tunnel. The anterior angle from the horizontal plane (X-axis) is represented as α = 35° (Reproduced with permission from Moatshe et al.).[57]](/content/115/2025/0/1/img/JASSM-63-2024-g011.png)
- To prevent tunnel convergence with the anterior cruciate ligament (ACL) reconstruction, the femoral tunnel for the fibular collateral ligament (FCL) should be drilled at a 35° anterior angle. When the patient is positioned supine, the surgeon lowers their hand while angling the reamer upward to achieve this trajectory. The tunnel for the popliteus tendon (PLT) is aligned parallel to the FCL tunnel. The anterior angle from the horizontal plane (X-axis) is represented as α = 35° (Reproduced with permission from Moatshe et al.).[57]
In lateral multi-ligament reconstructions, the FCL and PLT tunnels are at the highest risk of colliding with the single ACL tunnel.[60] For complex injuries involving both cruciate and collateral ligaments, where multiple tunnels are required in the distal femur, and proximal tibia, the risk of tunnel convergence is significantly elevated.[61] LaPrade et al. reported that for patients with combined ACL, PCL, and PLC injuries, reconstructing the three main PLC stabilizers using two allografts helps restore varus and rotational stability, especially in chronic cases.[17] Gursoy et al. also noted that special care is also needed when repairing meniscus root tears in conjunction with PLC reconstructions, as additional transtibial space is required.[62] Drilling the guide pins for the PLC tibial tunnel first allows for careful planning and potential adjustments to avoid convergence with other tunnels, maintaining structural integrity and optimal fixation.[60]
The presence of a proximal fibular fracture affects ligament reconstruction, which requires adjustments in tunnel placement to prevent fixation failure. If the fracture involves the fibular head, the fibular tunnel may need to be positioned more anteriorly or distally to avoid drilling through the fracture site. In cases where tunnels are contraindicated due to fracture instability, alternative fixation methods such as cortical buttons, suture anchors, or suspensory fixation should be considered.[63-68]
Post-operative rehabilitation
Post-PLC reconstruction, a structured rehabilitation protocol is essential for optimal recovery. During the first 6 weeks, the patient remains non-weight-bearing and wears a knee immobilizer, removed only for range of motion (ROM) exercises, dressing changes, and bathing.[1] Early rehabilitation focuses on restoring tibiofemoral and patellofemoral ROM, managing pain and edema, and reactivating the quadriceps.[4,69-71] ROM is initially limited to 0-90° of passive flexion for 2 weeks and gradually progresses to full flexion as tolerated.[4] At 6 weeks, patients may begin stationary cycling, start weaning off crutches, and transition from a knee immobilizer to a hinged knee brace, depending on quadriceps control.[4] Full weight-bearing allows progression to closed-chain strengthening exercises, initially focusing on muscular endurance before advancing to strength and power training. Resisted hamstring exercises are avoided for at least 4 months to protect the grafts.[1,72] Running and agility exercises are typically introduced around 6 months, depending on strength and power levels. Return to sports or high-demand activities occurs between 6 and 9 months once the affected knee demonstrates comparable strength, stability, and ROM to the unaffected side. Recovery timelines may vary if concurrent ligament injuries are addressed.[4]
Outcomes and assessment
Studies comparing PLC repair to reconstruction consistently favor reconstruction for better outcomes. Stannard et al.[53] and Levy et al.[51] reported higher reoperation rates for repairs, while Black and Stannard[73] found significantly lower failure rates with reconstruction. Stannard et al.[53] evaluated 56 patients with PLC injuries and reported a significantly higher failure rate for primary repair compared to ligament reconstruction (37% vs. 9%, P = 0.03). Moreover, reconstruction also resulted in better stability, with 64% of patients showing no varus laxity compared to 54% in the repair group. Although post-revision outcomes were similar, initial reconstruction reduced the need for revision surgery, further highlighting its superior long-term stability compared to primary repair. Similarly, Levy et al.[51] evaluated 83 patients with PLC injuries and reported a significantly higher failure rate for primary repair compared to reconstruction (40% vs. 6%, P < 0.001). In addition, reconstruction resulted in better stability with lower rates of residual varus and external rotation instability at follow-up. Finally, primary repair failures often require revision with reconstruction surgery, which further reinforces reconstruction as the preferred initial technique. Moreover, Black and Stannard[73] reviewed two high-quality comparative studies and found a significantly higher failure rate for repair compared to reconstruction (38.5% vs. 7.5%, P < 0.05). Despite similar Lysholm (86.5 vs. 89.5, P = 0.92) and IKDC subjective (70.4 vs. 68.6, P = 0.92) scores at final follow-up, the higher failure rate in repairs often required revision surgery. Further adding to the findings of previous studies, they emphasized that while primary repair may be an option in acute settings (<3 weeks post-injury) with good tissue quality, reconstruction remains the preferred technique due to its superior long-term stability and lower risk of complications.[73] Geeslin et al.[74] conducted a systematic review and reported IKDC scores ranging from 78.1 to 91.3 and Lysholm scores from 87.5 to 90.3 in patients treated within 3 weeks of injury. They also noted an overall failure rate of 19%, which increased to 38% in staged repairs.[74] Moulton et al.[18] reported a 90% success rate for chronic PLC injuries managed with reconstruction, as determined by varus stress testing and radiographic assessment. Postoperatively, Lysholm scores ranged from 65.5 to 91.8, with 9 out of 10 studies reporting scores above 80, which indicated favorable functional outcomes. Similarly, IKDC scores ranged from 62.6 to 86.0. Notably, 59% of patients had concomitant PCL injuries, while only 12% had isolated PLC injuries, which highlights the strong association between PLC instability and other ligamentous deficiencies. Despite variations in surgical techniques, the study emphasized that anatomic-based reconstructions that include fibular sling techniques and tibial tunnel-based approaches consistently yielded favorable outcomes.
Furthermore, the LaPrade anatomical reconstruction technique has demonstrated superior results, restoring stability and reducing laxity. Varus stress radiographs showed an improvement in laxity from a 6.2 mm pre-operative SSD to 0.1 mm at follow-up.[15] Patient-reported outcomes also improved significantly, with Cincinnati scores rising from 21.9 to 81.4 and IKDC subjective scores from 29.1 to 81.5.[5] These findings underscore the importance of timely anatomical reconstruction to achieve optimal recovery and prevent further knee degeneration.[1,4]
Although the LaPrade anatomical reconstruction technique has demonstrated superior outcomes in restoring PLC stability, certain aspects remain subject to debate. One notable concern is the role of the PFL in stabilizing the proximal tibiofibular (PTF) joint. The PFL plays a key role in external rotation restraint and varus stability; however, its direct contribution to proximal tibiofibular stabilization remains unclear. Some studies suggest that reconstructing the PFL in isolation may be insufficient to restore stability to the PTF joint, especially in cases where PTF instability coexists with PLC injuries.[75] In their study, Jabara et al. demonstrated a 9% incidence rate of PTF joint instability in the setting of multiligament knee injuries.[76] In addition, excessive tensioning of the reconstructed PFL could alter the biomechanics of the fibular head, potentially leading to altered joint kinematics or discomfort.[77] Further biomechanical research is warranted to determine whether additional stabilization techniques, such as fibular-based augmentations or alternative graft placements, are needed to optimize outcomes in patients with concomitant PLC and PTF joint instability.
While studies consistently favor reconstruction over repair for PLC injuries, several limitations must be considered when interpreting these findings. One major limitation is the variability in surgical techniques across studies, including differences in graft type (allograft vs. autograft), tunnel placement, and fixation methods. In addition, the heterogeneity in patient populations, particularly in the proportion of isolated PLC injuries compared to combined PLC-PCL or PLC-ACL injuries, further complicates the generalizability of the findings across studies.
Moreover, rehabilitation protocols also varied significantly among studies, with differences in postoperative weight-bearing restrictions, bracing protocols, and return-to-play criteria. This inconsistency in rehabilitation strategies may influence clinical outcomes and could contribute to differences in reported success rates. Another important limitation in the literature is the lack of high-level evidence, with most studies being retrospective cohort studies or case series (Level III and IV evidence). The absence of randomized controlled trials limits the ability to draw definitive conclusions regarding the superiority of reconstruction over repair. In addition, long-term outcomes beyond 5-10 years remain underreported, making it difficult to assess the durability of different surgical approaches over time. Despite these limitations, current evidence strongly supports reconstruction as the superior approach with lower failure and revision rates and better long-term stability compared to repair. However, future research should focus on standardizing surgical techniques and conducting high-quality prospective studies to further refine treatment strategies for PLC injuries. Moreover, standardization of rehabilitation protocols is another important area for improvement with prospective studies comparing accelerated rehabilitation programs to traditional staged approaches needed to determine the optimal strategy to improve recovery and improve long-term functional outcomes while minimizing complications such as graft failure and arthrofibrosis.
CONCLUSION
The FCL, PLT, and PFL are key stabilizers of the PLC, primarily resisting varus stress and external rotation while providing secondary support against anterior tibial translation. These injuries often occur with cruciate ligament tears and are frequently missed in acute settings. Over the past three decades, reconstruction has been shown to be superior to repair, with the early surgical intervention offering better outcomes. Anatomical reconstruction techniques, supported by biomechanical and clinical studies, have proven most effective in restoring knee stability and achieving optimal subjective and radiographic results at follow-up.
Author contributions
JEN, BS, LVT, RFL: Concept and design of the study; JEN, BS: Literature review; JEN, BS: Analysis and interpretation of literature; JEN, BS, LVT, RFL: Drafting of the article or revising, final approval, and accountable for all aspects.
Ethical approval
The Institutional Review Board approval is not required since it was a narrative review.
Declaration of patient consent
Patient’s consent not required as patients identity is not disclosed or compromised.
Conflicts of interest
Robert F. LaPrade reports a relationship with Ossur Americas that includes: consulting or advisory. Robert F. LaPrade reports a relationship with Smith and Nephew Inc that includes: consulting or advisory. Robert F. LaPrade reports a relationship with Linvatec Europe that includes: consulting or advisory. Robert F. LaPrade reports a relationship with Responsive Arthroscopy that includes: consulting or advisory. Robert F. LaPrade reports a relationship with Ossur Americas Inc that includes: funding grants. Robert F. LaPrade reports a relationship with Smith and Nephew Inc that includes: funding grants. Robert F. LaPrade reports a relationship with Arthroscopy Association of North America that includes: funding grants.
Robert F. LaPrade reports a relationship with American Orthopedic Society for Sports Medicine that includes: funding grants.
Use of artificial intelligence (AI)-assisted technology for manuscript preparation
The authors confirm that there was no use of artificial intelligence (AI)-assisted technology for assisting in the writing or editing of the manuscript and no images were manipulated using AI.
Financial support and sponsorship: Nil.
References
- The posterolateral corner: Explanations and outcomes. J Arthrosc Surg Sports Med. 2021;2:108-18.
- [CrossRef] [Google Scholar]
- Location of bone bruises and other osseous injuries associated with acute grade III isolated and combined posterolateral knee injuries. Am J Sports Med. 2010;38:2502-8.
- [CrossRef] [PubMed] [Google Scholar]
- A prospective magnetic resonance imaging study of the incidence of posterolateral and multiple ligament injuries in acute knee injuries presenting with a hemarthrosis. Arthroscopy. 2007;23:1341-7.
- [CrossRef] [PubMed] [Google Scholar]
- Posterolateral corner of the knee: Current concepts. Arch Bone Jt Surg. 2016;4:97-103.
- [Google Scholar]
- Biomechanical analysis of a posterior cruciate ligament reconstruction. Deficiency of the posterolateral structures as a cause of graft failure. Am J Sports Med. 2000;28:32-9.
- [CrossRef] [PubMed] [Google Scholar]
- The effects of grade III posterolateral knee complex injuries on anterior cruciate ligament graft force. A biomechanical analysis. Am J Sports Med. 1999;27:469-75.
- [CrossRef] [PubMed] [Google Scholar]
- The effect of injury to the posterolateral structures of the knee on force in a posterior cruciate ligament graft: A biomechanical study. Am J Sports Med. 2002;30:233-8.
- [CrossRef] [PubMed] [Google Scholar]
- Posterolateral corner injury In: Espregueira-Mendes J, Karlsson J, Musahl V, Ayeni OR, eds. Orthopaedic sports medicine: An encyclopedic review of diagnosis, prevention, and management. Berlin: Springer International Publishing; 2023. p. :1-19.
- [CrossRef] [Google Scholar]
- Evidence-based management of complex knee injuries e-book: Restoring the anatomy to achieve best outcomes Netherlands: Elsevier Health Sciences; 2020. p. :112-26.
- [Google Scholar]
- Posterolateral corner injuries: Epidemiology, anatomy, biomechanics and diagnosis. Injury. 2018;49:1024-31.
- [CrossRef] [PubMed] [Google Scholar]
- Treatment or acute and chronic combined anterior cruciate ligament and posterolateral knee ligament injuries. Sports Med Arthrosc Rev. 1997;5:91.
- [CrossRef] [Google Scholar]
- Posterolateral corner injuries of the knee: A serious injury commonly missed. J Bone Joint Surg Br. 2011;93:194-7.
- [CrossRef] [PubMed] [Google Scholar]
- Outcomes of untreated posterolateral knee injuries: An in vivo canine model. Knee Surg Sports Traumatol Arthrosc. 2011;19:1192-7.
- [CrossRef] [PubMed] [Google Scholar]
- Assessment of healing of grade III posterolateral corner injuries: An in vivo model. J Orthop Res. 2004;22:970-5.
- [CrossRef] [PubMed] [Google Scholar]
- Outcomes of treatment of acute grade-III isolated and combined posterolateral knee injuries: A prospective case series and surgical technique. J Bone Joint Surg Am. 2011;93:1672-83.
- [CrossRef] [PubMed] [Google Scholar]
- Improving outcomes for posterolateral knee injuries. J Orthop Res. 2014;32:485-91.
- [CrossRef] [PubMed] [Google Scholar]
- Outcomes of an anatomic posterolateral knee reconstruction. J Bone Joint Surg Am. 2010;92:16-22.
- [CrossRef] [PubMed] [Google Scholar]
- A systematic review of the outcomes of posterolateral corner knee injuries, part 2: Surgical treatment of chronic injuries. Am J Sports Med. 2016;44:1616-23.
- [CrossRef] [PubMed] [Google Scholar]
- Fibular collateral ligament anatomical reconstructions: A prospective outcomes study. Am J Sports Med. 2010;38:2005-11.
- [CrossRef] [PubMed] [Google Scholar]
- An analysis of an anatomical posterolateral knee reconstruction: An in vitro biomechanical study and development of a surgical technique. Am J Sports Med. 2004;32:1405-14.
- [CrossRef] [PubMed] [Google Scholar]
- The posterolateral attachments of the knee: A qualitative and quantitative morphologic analysis of the fibular collateral ligament, popliteus tendon, popliteofibular ligament, and lateral gastrocnemius tendon. Am J Sports Med. 2003;31:854-60.
- [CrossRef] [PubMed] [Google Scholar]
- Anatomy and biomechanics of the lateral side of the knee and surgical implications. Sports Med Arthrosc Rev. 2015;23:2-9.
- [CrossRef] [PubMed] [Google Scholar]
- The fibular collateral ligament-biceps femoris bursa. An anatomic study. Am J Sports Med. 1997;25:439-43.
- [CrossRef] [PubMed] [Google Scholar]
- Fibular collateral ligament: Varus stress radiographic analysis using 3 different clinical techniques. Orthop J Sports Med. 2018;6 Available from
- [CrossRef] [PubMed] [Google Scholar]
- Analysis of the static function of the popliteus tendon and evaluation of an anatomic reconstruction: The “fifth ligament” of the knee. Am J Sports Med. 2010;38:543-9.
- [CrossRef] [PubMed] [Google Scholar]
- Anatomy and biomechanics of the posterolateral corner of the knee. J Knee Surg. 2005;18:137-45.
- [CrossRef] [PubMed] [Google Scholar]
- Anatomic posterolateral knee reconstructions require a popliteofibular ligament reconstruction through a tibial tunnel. Am J Sports Med. 2010;38:1674-81.
- [CrossRef] [PubMed] [Google Scholar]
- Anatomic fibular collateral ligament reconstruction. Arthrosc Tech. 2016;5:e309-14.
- [CrossRef] [PubMed] [Google Scholar]
- A Comprehensive reanalysis of the distal iliotibial band: Quantitative anatomy, radiographic markers, and biomechanical properties. Am J Sports Med. 2017;45:2595-603.
- [CrossRef] [PubMed] [Google Scholar]
- The involvement of the anterolateral ligament in rotational control of the knee. Am J Sports Med. 2016;44:1209-14.
- [CrossRef] [PubMed] [Google Scholar]
- Classification of knee ligament instabilities. Part II. The lateral compartment. J Bone Joint Surg Am. 1976;58:173-9.
- [CrossRef] [PubMed] [Google Scholar]
- Posterolateral corner knee injuries: A narrative review. EFORT Open Rev. 2021;6:676-85.
- [CrossRef] [PubMed] [Google Scholar]
- Injuries to the posterolateral aspect of the knee. Association of anatomic injury patterns with clinical instability. Am J Sports Med. 1997;25:433-8.
- [CrossRef] [PubMed] [Google Scholar]
- Posterolateral corner injury of the knee: Evaluation and management. J Am Acad Orthop Surg. 2008;16:506-18.
- [CrossRef] [PubMed] [Google Scholar]
- Posterolateral corner injuries of the knee: Anatomy, diagnosis, and treatment. Sports Med Arthrosc Rev. 2006;14:213-20.
- [CrossRef] [PubMed] [Google Scholar]
- The role of the cruciate and posterolateral ligaments in stability of the knee. A biomechanical study. Am J Sports Med. 1995;23:436-43.
- [CrossRef] [PubMed] [Google Scholar]
- Evaluation of the reliability of the dial test for posterolateral rotatory instability: A cadaveric study using an isotonic rotation machine. Arthrosc J Arthrosc Relat Surg. 2008;24:593-8.
- [CrossRef] [PubMed] [Google Scholar]
- Medial collateral ligament injury of the knee: A review on current concept and management. Arch Bone Jt Surg. 2021;9:255-62.
- [Google Scholar]
- Tests for posterolateral instability of the knee in normal subjects. Results of examination under anesthesia. J Bone Joint Surg Am. 1991;73:30-6.
- [CrossRef] [Google Scholar]
- The external rotation recurvatum test revisited: Reevaluation of the sagittal plane tibiofemoral relationship. Am J Sports Med. 2008;36:709-12.
- [CrossRef] [PubMed] [Google Scholar]
- The posterolateral drawer test and external rotational recurvatum test for posterolateral rotatory instability of the knee. Clin Orthop. 1980;147:82-7.
- [CrossRef] [Google Scholar]
- The heel height test: A novel tool for the detection of combined anterior cruciate ligament and fibular collateral ligament tears. Arthrosc J Arthrosc Relat Surg. 2017;33:2177-81.
- [CrossRef] [PubMed] [Google Scholar]
- The reproducibility and repeatability of varus stress radiographs in the assessment of isolated fibular collateral ligament and grade-III posterolateral knee injuries. An in vitro biomechanical study. J Bone Joint Surg Am. 2008;90:2069-76.
- [CrossRef] [PubMed] [Google Scholar]
- Diagnosis and treatment of multiligament knee injury: State of the art. J ISAKOS. 2017;2:152-61.
- [CrossRef] [Google Scholar]
- Increased accuracy of varus stress radiographs versus magnetic resonance imaging in diagnosing fibular collateral ligament grade III tears. Arthrosc J Arthrosc Relat Surg. 2018;34:2230-5.
- [CrossRef] [PubMed] [Google Scholar]
- Correlation between magnetic resonance imaging and physical exam in assessment of injuries to posterolateral corner of the knee. Acta Ortop Bras. 2014;22:124.
- [CrossRef] [PubMed] [Google Scholar]
- Posterolateral complex knee injuries: Magnetic resonance imaging with surgical correlation. Acta Radiol Stockh Swed. 1987-2005;46:297-305.
- [CrossRef] [PubMed] [Google Scholar]
- Nonoperative treatment of grade II and III sprains of the lateral ligament compartment of the knee. Am J Sports Med. 1989;17:83-8.
- [CrossRef] [PubMed] [Google Scholar]
- Lateral ligament injuries of the knee. Knee Surg Sports Traumatol Arthrosc. 1998;6:21-5.
- [CrossRef] [PubMed] [Google Scholar]
- Combined posterior cruciate ligament injuries. Clin Sports Med. 1994;13:629-47.
- [CrossRef] [Google Scholar]
- Repair versus reconstruction of the fibular collateral ligament and posterolateral corner in the multiligament-injured knee. Am J Sports Med. 2010;38:804-9.
- [CrossRef] [PubMed] [Google Scholar]
- Insall & Scott Surgery of the Knee. 2011. Available from: https://shop.elsevier.com/books/insall-and-scott-surgery-of-the-knee/scott/978-1-4377-1503-3 [Last accessed on 2024 Nov 10]
- [Google Scholar]
- The posterolateral corner of the knee: Repair versus reconstruction. Am J Sports Med. 2005;33:881-8.
- [CrossRef] [PubMed] [Google Scholar]
- Double-row suture anchor repair of posterolateral corner avulsion fractures. Arthrosc Tech. 2017;6:e997-1001.
- [CrossRef] [PubMed] [Google Scholar]
- Strategies for preventing tunnel convergence in multiligament knee injury reconstructions. Indian J Orthop. 2024;58:1528-36.
- [CrossRef] [PubMed] [Google Scholar]
- A hamstring-based anatomic posterolateral knee reconstruction with autografts improves both radiographic instability and functional outcomes. Arthrosc J Arthrosc Relat Surg. 2019;35:1676-85.e3.
- [CrossRef] [PubMed] [Google Scholar]
- Multiple ligament reconstruction femoral tunnels: Intertunnel relationships and guidelines to avoid convergence. Am J Sports Med. 2017;45:563-9.
- [CrossRef] [PubMed] [Google Scholar]
- Intertunnel relationships in the tibia during reconstruction of multiple knee ligaments: How to avoid tunnel convergence. Am J Sports Med. 2016;44:2864-9.
- [CrossRef] [PubMed] [Google Scholar]
- How to avoid tunnel convergence in a multiligament injured knee. Ann Jt. 2018;3:93.
- [CrossRef] [Google Scholar]
- Multiple ligament reconstructions of the knee and posterolateral corner. Arthrosc Tech. 2021;10:e1269-80.
- [CrossRef] [PubMed] [Google Scholar]
- Reduction of collision risk in multi-ligament knee injury KD-III-M and KD-IV surgery-superficial medial collateral ligament reconstruction with suture anchors. Arthrosc Tech. 2023;12:e413-20.
- [CrossRef] [PubMed] [Google Scholar]
- Optimal tibial tunnel placement for medial and lateral meniscus root repair on the anteromedial tibia in the setting of anterior and posterior cruciate ligament reconstruction of the knee. Am J Sports Med. 2022;50:1237-44.
- [CrossRef] [PubMed] [Google Scholar]
- Cortical button fixation for proximal tibiofibular instability: A technical report. Arthrosc Tech. 2020;9:e1415-21.
- [CrossRef] [PubMed] [Google Scholar]
- Proximal tibiofibular joint instability and treatment approaches: A systematic review of the literature. Arthrosc J Arthrosc Relat Surg. 2017;33:1743-51.
- [CrossRef] [PubMed] [Google Scholar]
- Syndesmosis stabilisation: Screws versus flexible fixation. Foot Ankle Clin. 2017;22:35-63.
- [CrossRef] [PubMed] [Google Scholar]
- Suture-button syndesmosis fixation: Accelerated rehabilitation and improved outcomes. Clin Orthop. 2005;431:207-12.
- [CrossRef] [Google Scholar]
- To compare the efficacy between fixation with tightrope and screw in the treatment of syndesmotic injuries: A meta-analysis. Foot Ankle Surg. 2019;25:63-70.
- [CrossRef] [PubMed] [Google Scholar]
- Improved reduction of the tibiofibular syndesmosis with tightrope compared with screw fixation: Results of a randomized controlled study. J Orthop Trauma. 2019;33:531-7.
- [CrossRef] [PubMed] [Google Scholar]
- Loss of normal knee motion after anterior cruciate ligament reconstruction is associated with radiographic arthritic changes after surgery. Am J Sports Med. 2012;40:108-13.
- [CrossRef] [PubMed] [Google Scholar]
- Clinic-based patellar mobilization therapy for knee osteoarthritis: A randomized clinical trial. Ann Fam Med. 2018;16:521-9.
- [CrossRef] [PubMed] [Google Scholar]
- Minimum 10-year results after anterior cruciate ligament reconstruction: How the loss of normal knee motion compounds other factors related to the development of osteoarthritis after surgery. Am J Sports Med. 2009;37:471-80.
- [CrossRef] [PubMed] [Google Scholar]
- Tendon healing: An overview of physiology, biology, and pathology of tendon healing and systematic review of state of the art in tendon bioengineering. Knee Surg Sports Traumatol Arthrosc. 2015;23:2097-105.
- [CrossRef] [PubMed] [Google Scholar]
- Repair versus reconstruction in acute posterolateral instability of the knee. Sports Med Arthrosc Rev. 2015;23:22-6.
- [CrossRef] [PubMed] [Google Scholar]
- A systematic review of the outcomes of posterolateral corner knee injuries, part 1: Surgical treatment of acute injuries. Am J Sports Med. 2016;44:1336-42.
- [CrossRef] [PubMed] [Google Scholar]
- Anatomic reconstruction of the proximal tibiofibular joint. Arthrosc Tech. 2016;5:e207-10.
- [CrossRef] [PubMed] [Google Scholar]
- Is stability of the proximal tibiofibular joint important in the multiligament-injured knee? Clin Orthop. 2014;472:2691-7.
- [CrossRef] [PubMed] [Google Scholar]
- Reconstruction of the posterolateral corner of the knee In: Doral MN, Karlsson J, eds. Sports injuries: Prevention, diagnosis, treatment and rehabilitation. Berlin: Springer; 2020. p. :1-20.
- [CrossRef] [Google Scholar]