Translate this page into:
Does retensioning of adjustable-loop cortical suspension devices improve performance: A systematic review and meta-analysis
*Corresponding author: Seth Theodore Campbell, Department of Orthopedic Surgery, Mclaren Flint, Flint, Michigan, United States. sethcampbell2012@gmail.com
-
Received: ,
Accepted: ,
How to cite this article: Campbell ST, Liu J, Gowd AK, Amin N, Sardelli M, Morrison M. Does retensioning of adjustable-loop cortical suspension devices improve performance: A systematic review and meta-analysis. J Arthrosc Surg Sports Med. 2024;5:3-11. doi: 10.25259/JASSM_22_2022
Abstract
Objectives:
To date, there is conflicting evidence when comparing fixed-loop cortical suspension devices (FLDs) to adjustable-loop devices (ALDs). Some studies indicate that ALDs are inferior to FLD in regard to displacement and failure load while others show that they are biomechanically similar. The purpose of this study is to use a meta-analysis of biomechanical data to compare FLDs to ALDs with and without retensioning. It is hypothesized that retensioning the ALD will allow these devices to be biomechanically equivalent to the FLD in total unloaded displacement and failure load.
Materials and Methods:
This study sought to identify all biomechanical studies that compared fixed loops to ALD. A meta-analysis was performed to find the standardized mean difference with retensioning as a covariate.
Results:
The analysis of isolated tests showed that retensioning reduced the cyclic ALD displacement in comparison to non-retensioned ALD; however, both the ALD with and without retensioning had significantly higher cyclical displacement and significantly lower failure load compared to the FLD. In the meta-analysis of the animal model data, there was no significant difference between the ALD with retensioning and the FLD.
Conclusion:
This analysis suggests that retensioning reduces displacement of an ALD, with displacements measured in animal bone testing showing no significant difference as compared to FLD. However, in the device-only model, the ALD with retensioning and the ALD without retensioning had significantly higher cyclical displacement and significantly lower load to failure compared to the FLD. These data suggest that retensioning may be beneficial. However, there is significant heterogeneity in the pooled studies which limit the strength of this conclusion.
Keywords
Anterior cruciate ligament
Cortical suspension device
Adjustable loop
Biomechanical
INTRODUCTION
In the United States, alone it is estimated that there are approximately 130,000 anterior cruciate ligament (ACL) reconstruction surgeries annually.[1] There are many techniques used for graft fixation: cortical suspension devices, interference screws, and cross-pin devices, with each having advantages and disadvantages.[2,3] Due to concerns over the biomechanical strength of the interference screws[4,5] and the operative complications of the cross-pin devices,[2,6,7] other methods of fixation, such as cortical suspension devices, have been developed to optimize the biomechanical and insertional qualities.
Two main categories of cortical suspension devices are the fixed-loop device (FLD) and the adjustable-loop device (ALD). FLD can provide biomechanically sound fixation with minimal elongation as the graft incorporates into the bone. However, these devices also require precise calculations regarding the graft and tunnel length, and they do not permit change in length when encountering differing bone tunnel dimensions.[8] ALD allows for simpler insertion by avoiding intraoperative calculations as well as minimizing over-drilling,[9] which maximizes the bone-graft interface. There are concerns, however, that these devices may exhibit increased displacement with cyclical loading when compared to FLD-type devices in some biomechanical studies.[2,3,9-15]
To date, the literature has provided conflicting evidence when comparing FLDs and ALDs. Some biomechanical studies concluded that the FLD showed less total displacement as well as higher ultimate failure loads.[2,3,9-14] Other biomechanical studies have concluded that fixed and ALD are biomechanically similar in total displacement as well as failure load.[8,16-18] One of the unique benefits of an ALD is the ability to re-tension after intraoperative cyclical loading, which allows the surgeon to retighten the device and remove some of the initial laxity. Few studies, including a recent meta-analysis,[15] have evaluated the effects of retensioning in their analysis. There is some evidence to suggest that retensioning the ALD could possibly make the ALD biomechanically similar to the FLD,[8,13,17,18] while a single study concluded that it made no difference.[13]
The purpose of this study is to compare FLDs to ALDs with and without retensioning to determine whether retensioning the device can recapture some displacement and make it biomechanically equivalent to the FLD. It is hypothesized that retensioning the ALD would allow these devices to be biomechanically equivalent to the FLD in total unloaded displacement and failure load.
MATERIALS AND METHODS
Search criteria and data extraction
PubMed, Embase, and Cochrane Library databases were searched for eligible studies up to July 15, 2020, using the search terms (cortical or suspensory) and “anterior cruciate ligament” and “biomechanical.” Resulting abstracts were reviewed to identify any biomechanical model studies that compared adjustable-loop systems to fixed-looped systems and tested for displacement and or load failure were included for analysis. Any studies that published retractions or studies that were challenged in published letters to the editor were excluded from the study.
Included studies were examined to identify the primary outcomes of interest which were the total displacement (in mm) and failure load (in N) of each device. In cases where the data of interest were provided graphically (rather than in the text or a table), the authors were contacted to request the study data. In these cases where the authors did not respond (two cases) an image analysis tool (ImageJ, NIH) was used to digitally extract the data.[14,19] In cases where data were reported as median/range, the method of Hozo et al.[20] was used to convert the data into estimated mean and standard deviation. Rylander et al. did not evaluate for displacement using methods similar to the other studies and so the displacement data from that study was not included in the analysis.[21] Study methods were also extracted and documented in tables.
Quality/bias assessment
The methodological quality/risk of bias of the selected articles was assessed independently by two reviewers using a standard checklist and scoring method [Table SI 1].[22] Briefly, each article was evaluated for five items with each scored as 0 if well addressed, 1 if partially addressed/unclear, and 2 if not addressed. The assessment items focused on (1) sampling methods for the devices included, (2) clarity and consistency in testing protocol, (3) sample size justification, (4) clarity and consistency in data analysis protocol, and (5) complete reporting of data. A total quality score was calculated and articles scoring 0 < 3 were assigned a grade low risk of bias, 4–7 rated moderate risk, and 8 or higher rated high risk. Where there was a discrepancy between the reviewer’s ratings, the score was discussed to arrive at a consensus.
Statistical analysis
Data from device-only models (DOMs) were analyzed separately from data gathered using animal models to limit heterogeneity in the testing methods of each study, as demonstrated in Kamelger et al.’s[14] study which showed significant differences between results from these testing modes. Statistical analysis was performed using the open-source software OpenMeta (http://www.cebm.brown.edu/openmeta/) and SigmaStat (Systat Software Inc. San Jose California USA).
The primary analysis was a sub-group meta-analysis to examine the within-study differences between ALD and FLD. In this analysis, the subgrouping was based on whether the ADL was retensioned or not. The standardized mean difference and 95th confidence intervals (CIs) for each group were found using a random effects model.[8] In studies where there were multiple control devices tested, ALDs were matched to FLD using a random assignment process. A secondary meta-analysis grouped all devices into three groups (grouping data across studies): the FLD (Group 1), the ALD without retensioning (Group 2), and the ALD with retensioning (Group 3).
For all meta-analyses, a Wald test was performed to determine if the standardized mean difference for each group (for the within-study difference analysis) or the group means were non-zero. Forest plots were created for each analysis and the I2 index was used to measure the heterogeneity of included studies.[23] Index scores <25% are considered to indicate low heterogeneity, 20–50% moderate, and >75% considered high.[24] Secondary analyses to identify significant differences between retensioned and not retensioned ADL pooled means from the meta-analysis of within study differences utilized t-tests. Analysis of variance analyses with Holm-Sidak post hoc testing were utilized in comparing data pooled across studies for Groups 1–3. All analyses were done with a 95% CI.
RESULTS
Using the above search criteria, a total of 274 studies were identified in the search. Among these 33 full-text articles were assessed for eligibility [Figure 1]. Within these 18 studies were excluded after review, leaving 15 final studies included in the study.
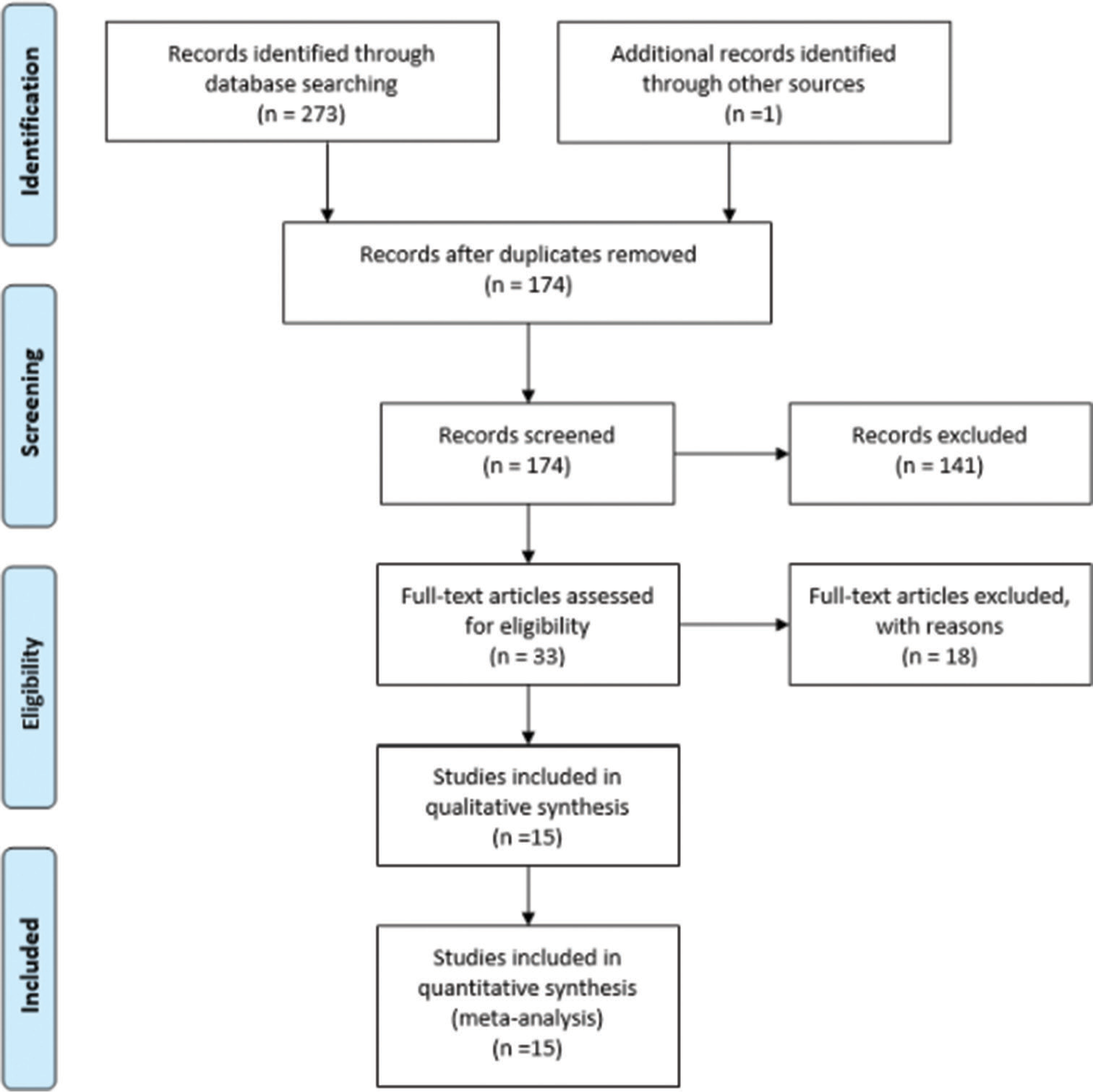
- Search strategy using the preferred reporting items for systematic reviews and meta-analyses guidelines. n=Number of studies.
The included studies involved a range of devices with the most common control being the ENDOBUTTON™ (Smith and Nephew, Memphis, TN) and the most common adjustable devices being the TightRope® RT (TRT, Arthrex, Naples, FL) and ToggleLoc™ Device with ZipLoop® Technology (TLZ; Zimmer Biomet, Warsaw, IN) [Table 1].
![]() |
N/A means that it was not detailed in the study. mm: Millimeters, min: Minute, s: Second, Hz: Hertz, N: Newtons
None of the included studies were determined to present a high risk of bias. Three studies[9,14,17] were assessed to have moderate potential for bias based on a missing or poorly documented sample size calculation and lack of documentation/potentially non-random sample selection. Please refer to the online only Supplementary Information (SI), [Table SI 1] for the score for each paper.
Experimental setup
The setup for the “device-only model” studies involved testing the devices in isolation. These studies typically passed the device through a hole in a steel plate rather than bone. After feeding the button through the opening, the button was secured against the plate surface simulating a lateral femoral cortex. The free end of the loop was then put over a rod or a hook. The loop lengths remained equal for both the ALD and FLD in each study but differed between studies.
In the tests utilizing animal bone models (ABMs), bovine tendons were cut to a certain length, then doubled or quadrupled over and sutured together to replicate an ACL construct. Graft measurements were taken to size the bone tunnel. The femoral tunnels were drilled to various distances with a reamer leaving a varying amount of bone bridge at the cortex. The tunnel length is the distance of larger diameter tunnel for the graft. The graft with the attached loop device was pulled through the femoral tunnel, pulled through the smaller diameter tunnel until the button was on the cortex, and the button was then flipped. The ALDs were, then, adjusted so that the graft filled the tunnel. Götschi et al.[25] attached a tendon graft to the device without using animal bone. Smith et al.[8] used both a femur and a tibia in their animal model with the cortical button fixation on the femoral side and an interference screw on the tibia side. However, in their TightRope® RT group, an all-inside technique was used with an ALD on both the femur and tibial side.[8]
Most studies (DOM and ABM) precycled its construct. During this phase, lower forces for lower amounts of cycles were used to remove any slack from the setup and simulate intraoperative graft cycling. Any low load residual displacement after this was set to zero as the baseline. The total displacement value was recorded after subsequent higher load the cyclical loading. Loading levels and numbers of cycles in each study are shown in [Table 1]. After the cyclical loading protocol, failure testing would begin by pulling the setup by a certain distance per unit time until the setup would fail, this would be recorded as the failure load force.
Five studies retensioned their ALD but only three studies compared a retensioned device to a non-retensioned device. Johnson et al.,[13] Noonan et al.,[17] Singh et al.,[26] and Nye et al.[18] retensioned by pulling on the sutures after precycling. Smith et al.[8] used 200 N of force, or maximal force if 200 N could not be reached, to re-tension the femoral side. Two studies tied a knot on the outside of the adjustable loop.[10,17] The groups that had a knot but were not retensioned were analyzed in the non-retensioned group.
Meta-analysis of total displacement
The data extracted for the displacement analysis can be found on-line in the SI Data Source Tables ([Table SI 2a] for DOM displacements and [Table SI 2b] for ABM displacements).
In the DOM displacement analysis, the ALD with retensioning and the ALD without retensioning were both found to have significantly higher displacement values than the FLD (P < 0.002). When comparing the retensioned ALD to the non-retensioned ALD, there was no significant difference (P = 0.130) [Figure 2].
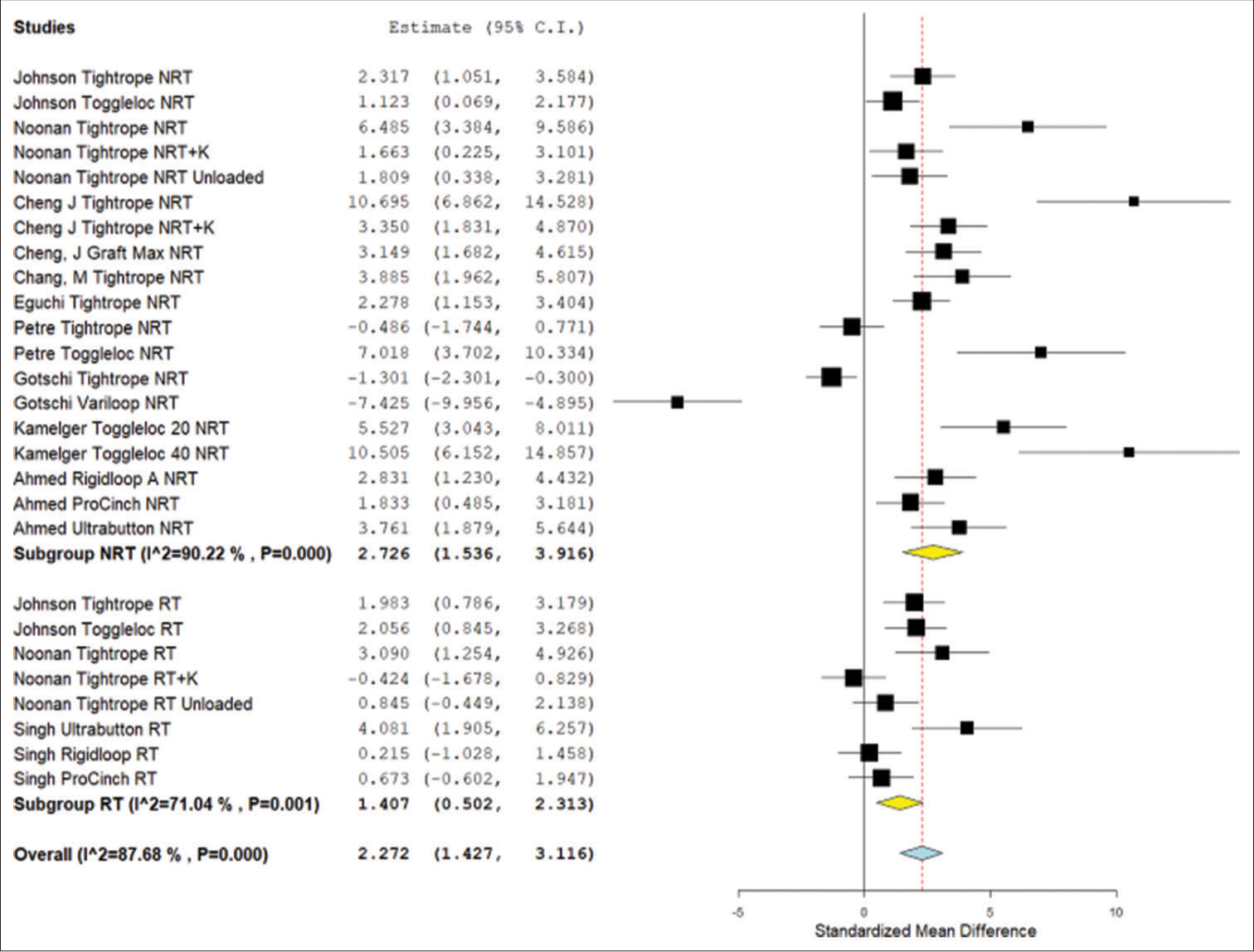
- Forest plot for the meta-analysis results of standard mean displacement difference for the device only model studies. NRT: Not retensioned, RT: Retensioned, K: Knotted. Unloaded refers to the Noonan et al. protocol that featured smaller lower limit forces during cyclical testing. Number “20” or “40” next to device refers to the length of the device loop in millimeters. RIGIDLOOP® A refers to adjustable RIGIDLOOP® device. RIGIDLOOP® NA refers to non-adjustable RIGIDLOOP® device. Yellow is the subgroup standardized mean difference. Blue is combined standardized mean difference. Black squares refer to the mean for that study and group. CI: Confidence Interval
In the ABM displacement analysis, there was a significant difference between the non-retensioned ALD and the FLD (P = 0.018). There was no significant difference between the retensioned ALD and the FLD (P = 0.995). When comparing the non-retensioned ALD to the retensioned ALD, there was no significant difference (P = 0.317) [Figure 3].
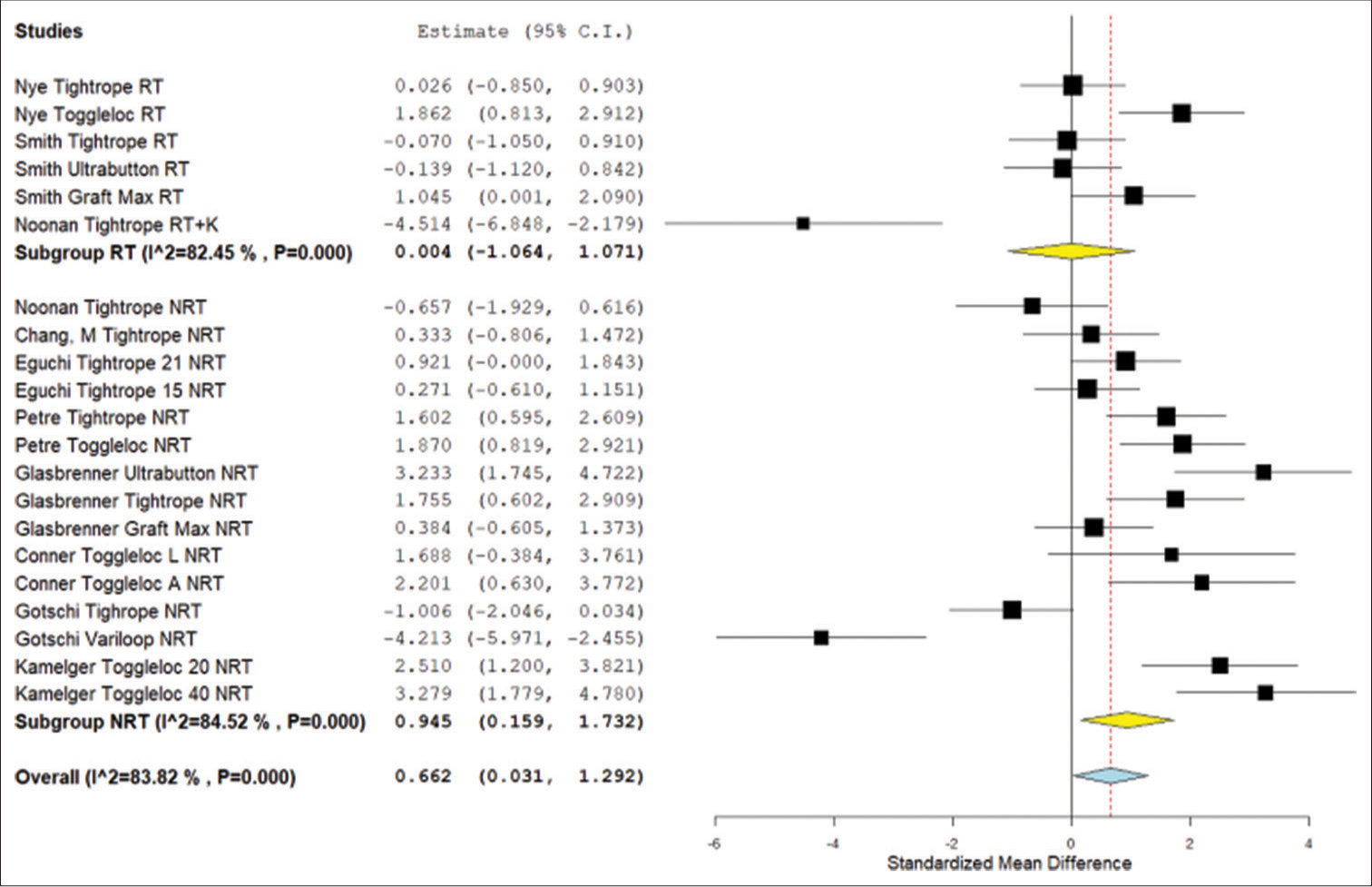
- Forest plot for the meta-analysis of standardized mean displacement difference from animal model studies. NRT: Not retensioned, RT: Retensioned, K: Knotted. Unloaded refers to the Noonan et al. protocol that featured smaller lower limit forces during cyclical testing. Number “15,” “20,” “21,” or “40” next to device refers to the length of the device loop in millimeters. “L” refers to placement on the lateral cortex whereas “A” refers to placement on the anterior cortex. Yellow is the subgroup standardized mean difference. Blue is combined standardized mean difference. Black squares refer to the mean for that study and group. CI: Confidence Interval.
The secondary across-study meta-analysis of total displacement for the three groups (ALD-retensioned, ALDnot retensioned, and FLD) yielded similar results to those from the standardized mean differences. Those data are available online in the SI [Figure SI 1].
Meta-analysis failure load
The data extracted for the failure load analysis can be found on-line in the SI Data Source Tables ([Table SI 3a] for DOM failure load and [Table SI 3b] for ABM failure load).
In the DOM failure load analysis, the ALD with retensioning and the ALD without retensioning both had significantly lower load to failure compared to the FLD (P < 0.003). There was no significant difference between the ALD without retensioning and the ALD with retensioning (P = 0.716) [Figure 4].
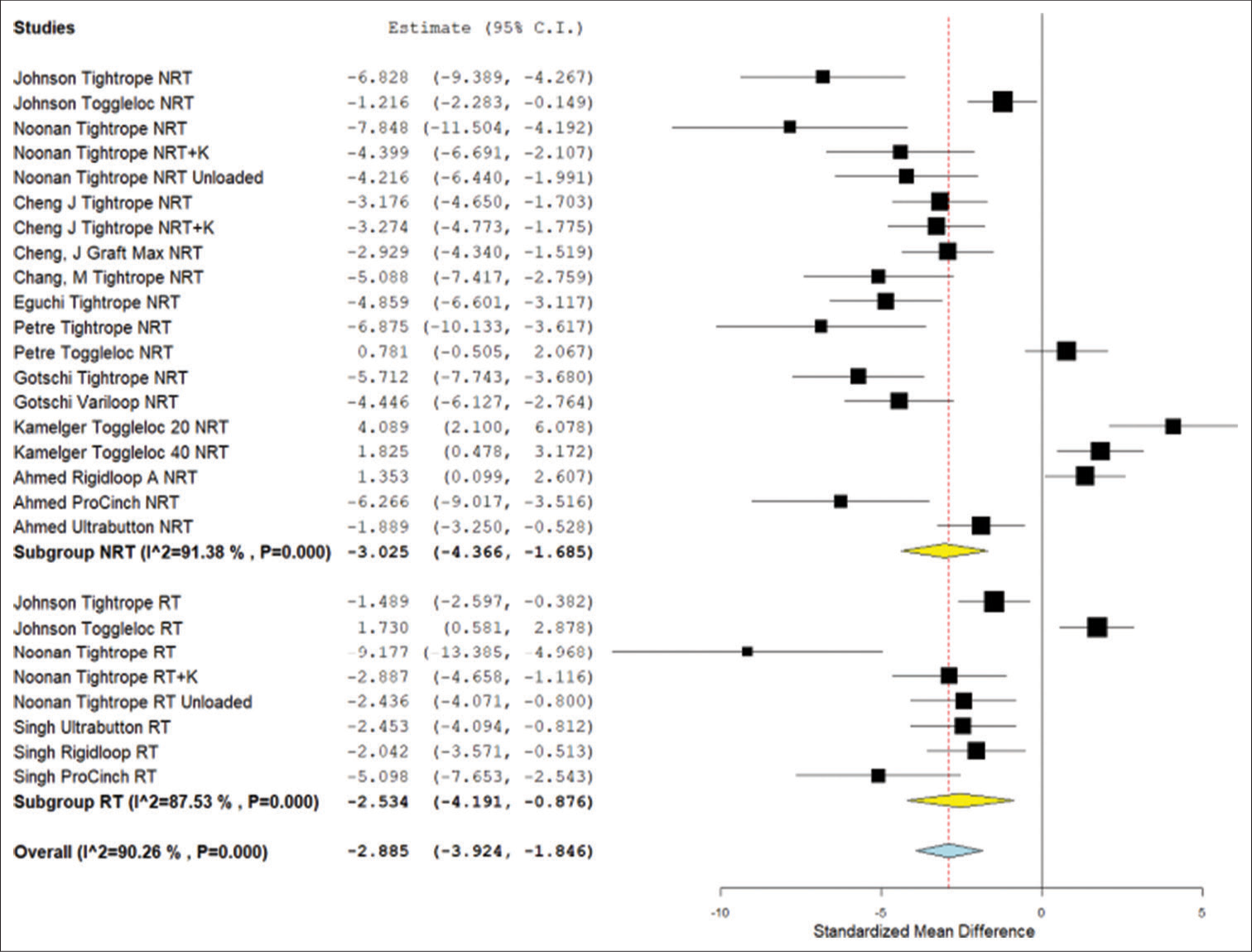
- Forest plot for standardized mean difference in failure load results from device only model studies. NRT: Not retensioned, RT: Retensioned, K: Knotted. Unloaded refers to the Noonan et al. protocol that featured smaller lower limit forces during cyclical testing. Number “20” or “40” next to device refers to length of device loop in millimeters. RIGIDLOOP® A refers to adjustable RIGIDLOOP® device. RIGIDLOOP® NA refers to non-adjustable RIGIDLOOP® device. Yellow is the subgroup standardized mean difference. Blue is combined standardized mean difference. Black squares refer to the mean for that study and group. CI: Confidence interval.
In the ABM failure load analysis, there was no significant difference between the ALD with retensioning and the FLD (P = 0.500). The ALD without retensioning had a significantly lower load to failure than the FLD (P < 0.001) and the ALD with retensioning (P = 0.008) [Figure 5].
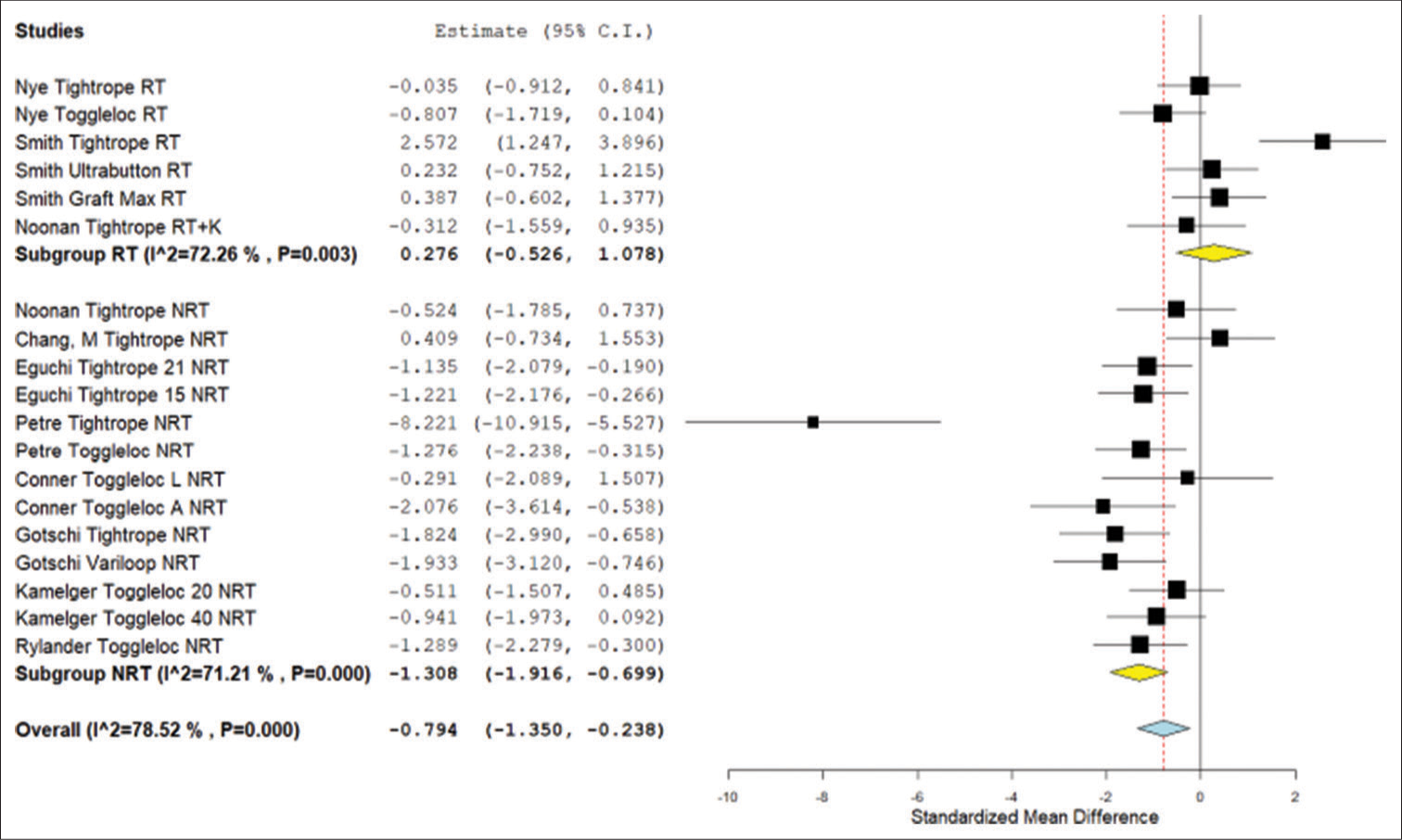
- Forest plot of the meta-analysis results for standardized mean difference in failure load in animal model studies. NRT: Not retensioned, RT: Retensioned, K: Knotted. Unloaded refers to the Noonan et al. protocol that featured smaller lower limit forces during cyclical testing. Number “15,” “20,” “21,” or “40” next to device refers to the length of the device loop in millimeters. “L” refers to placement on the lateral cortex whereas “A” refers to placement on the anterior cortex. Yellow is the subgroup standardized mean difference. Blue is combined standardized mean difference. Black squares refer to the mean for that study and group. CI: Confidence interval
The secondary across-study meta-analysis of failure forces for the three groups (ALD-retensioned, ALD-not retensioned, and FLD) yielded similar results to those from the standardized mean differences. Those data are available online in the SI [Figure SI 2].
DISCUSSION
This study demonstrated that isolated testing of both the ALD with retensioning and the ALD without retensioning had significantly higher cyclical displacement and significantly lower load to failure compared to the FLD. However, in the animal model data, there was no significant difference between the ALD with retensioning and the FLD. Meta-analyses regarding non-retensioned ALD demonstrated the higher displacement and the lower load to failure in comparison to FLD. The results of this study suggest that the use of an ALD with intraoperative retensioning may produce a biomechanically equivalent construct to that of the FLD.
The outcomes of this analysis compare favorably and expand on the results of a recent meta-analysis.[15] Houck et al. performed a meta-analysis comparing adjustable loops to fixed loops and found the adjustable loop had significantly higher displacement.[15] In contrast to this analysis, Houck et al. found the ENDOBUTTON™ FLD to have a similar load to failure as the ToggleLoc™ adjustable loop but a significantly higher load to failure compared to the TightRope® RT adjustable loop.[15] There have been concerns about the Houck study regarding the grouping of animal and DOMs as well as the exclusions of some biomechanical studies.[27] In addition, their study did not consider the retensioning of the adjustable loop. The present study separates the comparison of animal and device only testing and includes a larger number of studies. This analysis demonstrates the high variability in the data even with this separation, with heterogeneity scores above 70% for all analyses. This may be due to differences in the devices themselves or the testing protocols (e.g., peak load level during the cyclic loading regime).
Retensioning is an important property of the ALD and is recommended by multiple manufacturers.[28,29] After the precycling period, the sutures can be pulled tight again to restore some of the displacement caused during precycling. An initial displacement of 0.75 mm after one pull at 250 N of force was reported by Petre et al.[3] This displacement was 68% of the final lengthening seen after all the loading cycles were completed. When retensioning was done after the initial precycling, this reduced the initial length and, thus, the overall final displacement was significantly reduced.[17] Noonan et al.,[17] Nye et al.,[18] and Smith et al.[8] found that there was no significant difference in displacement between the FLD and the ALD that were retensioned. Conversely, Johnson et al.[13] found that the ALD with retensioning displaced significantly more than the FLD. This study provides further evidence to support retensioning. In the current study, there was no significant difference between the FLD and the ALD with retensioning in the animal model analysis. In contrast, there was a significant difference between the FLD and ALD with retensioning in the DOM. The animal model is more likely to be clinically relevant as the porcine bone used in the included studies has been shown to closely replicate the biomechanical properties of the human knee.[3,30,31] This analysis is also limited by the high heterogeneity of the studies.
Tying the free suture ends of the adjustable loop is another strategy that may be used to limit the cyclical displacement. There are two main adjustable-loop designs. One design uses a finger trap mechanism to prevent slippage once tension is applied while the other design uses a suture loop that locks once tension is applied. Once the button is retensioned after cyclical displacement, a knot can be tied to prevent the suture from sliding through the finger trap mechanism, or from slipping through the locking loop. This would create the equivalent of a fixed-loop construct. The evidence regarding the use of knotting is favorable but limited. Barrow et al.[2] and Noonan et al.[17] found that after securing the loose suture ends with knots that the adjustable loop displaced significantly less. Cheng et al.[10] found no significant difference in displacement after knotting the ALD. In regard to failure load, Barrow et al.[2] and Cheng et al.[10] found that knotting made no difference, while Noonan et al.[17] found that knotting significantly increased the failure load. This meta-analysis did not further investigate the utility of knotting the suture ends given the limited number of studies that studied this technique specifically.
Despite the findings of this biomechanical analysis, there are data suggesting that ALD and FLD have similar clinical outcomes. ACL clinical failure is defined as absolute displacement >10 mm or >3 mm of side to side difference, which is reflected by total displacement measures.[32] The cortical suspension device is supposed to maintain sufficient fixation so that graft tension is maintained until it is incorporated into native bone.[2,33,34] If the suspension device lengthens during this initial period, then there would be a loss of graft tension which may also lead to tunnel widening and impaired healing.[2,35] It should be cautioned that the additive effects of using dual suspensory fixation could lead to clinical failure. Smith et al, the only biomechanical study to evaluate dual suspensory ALD, found that it displaced more than using a single ALD, but did not reach statistical significance.[8] Supplementary tibial fixation, particularly in dual suspensory constructs, could be another method to potentially decrease cyclical elongation.[36] Both the ALD and FLD demonstrated higher load to failure than what is thought to occur during rehabilitation. The forces that load the device during early rehabilitation and ambulation have been shown to be between 300 N and 590 N,[6,14,33] while the grafts themselves have all been shown to be stronger than the native ACL with ultimate failure loads over 2300 N.[37] Therefore, because all three groups showed failure loads above 590 N, it is probable that they are strong enough to withstand the stress of early rehabilitation. In support of this, two recent systematic reviews of clinical studies found no clinical differences between the retensioned ALD and the FLD.[38,39] This discrepancy between biomechanical and clinical results could be partially explained by retensioning. Onggo et al.[38] noted that all the ALD used in clinical studies were retensioned compared to few retensioned ALD in biomechanical studies. Other possible factors for the discrepancy between biomechanical and clinical studies include differing loading angles and the effect of the graft healing in the setting of early rehabilitation.[38]
Limitations of the present study include the experimental setup of studies. These animal studies typically used forces that were in line with the bone tunnels rather than the force vectors that might be acting on the knee in vivo. In biomechanical studies, the loading force is placed solely on the device. Conversely, in the native knee, the forces applied to the devices are not purely linear, with the graft-bone interface sharing the loading force.[3,8,14] This means that the device most likely experiences lower forces in vivo and may not require as much strength as in vitro. The models lacked synovial fluid and tissue healing and were performed at time zero, which negates the effects of bone graft integration. The animal model consisted of fewer studies and could be underpowered to discover a significant difference. The heterogeneity of studies included is also a significant limitation. The I2 values of our studies ranged from 71% to 90%. This meta-analysis combined data from studies comparing a range of ALD and FLD designs, assuming that these devices respond similarly which may not be the case. The studies also differed in their lengths of devices, cycling protocols, bone tunnel lengths, and graft lengths as well. These results may not translate clinically and require further clinical study.
CONCLUSION
Retensioning ALD improved their biomechanical performance in animal models but did not significantly improve their performance during device-only testing. However, there is significant heterogeneity in the pooled biomechanical results. Establishing a consistent testing protocol for these devices may help show whether retensioning of ALD alters the biomechanical response for these devices.
Acknowledgments
The authors gratefully acknowledge Theresa Atkinson, PhD for her assistance in the data analysis and manuscript preparation.
Ethical approval
The research/study complied with the Helsinki Declaration of 1964.
Declaration of patient consent
Patient’s consent not required as there are no patients in this study.
Conflicts of interest
One or more of the authors has declared the following potential conflict of interest or source of funding: N.H.A. has received consulting fees from Pacira Pharmaceuticals, Smith and Nephew, Baudax Bio, AcelRx Pharmaceuticals, Trice Medical, Medical Device Business Services, Bioventus, KCI USA, and DePuy Synthes Products; royalties from Smith and Nephew; and hospitality payments from PAVmed and Acumed. J.N.L. has received support for education from Empire Medical, Smith and Nephew, and Arthrex and hospitality payments from Stryker Corporation and Smith and Nephew.
Use of artificial intelligence (AI)-assisted technology for manuscript preparation
The author(s) confirms that there was no use of artificial intelligence (AI)-assisted technology for assisting in the writing or editing of the manuscript and no images were manipulated using AI.
Financial support and sponsorship
Nil.
References
- Incidence and trends of anterior cruciate ligament reconstruction in the United States. Am J Sports Med. 2014;42:2363-70.
- [CrossRef] [PubMed] [Google Scholar]
- Femoral suspension devices for anterior cruciate ligament reconstruction: Do adjustable loops lengthen? Am J Sports Med. 2014;42:343-9.
- [CrossRef] [PubMed] [Google Scholar]
- Femoral cortical suspension devices for soft tissue anterior cruciate ligament reconstruction: A comparative biomechanical study. Am J Sports Med. 2013;41:416-22.
- [CrossRef] [PubMed] [Google Scholar]
- A comparison between a retrograde interference screw, suture button, and combined fixation on the tibial side in an all-inside anterior cruciate ligament reconstruction: A biomechanical study in a porcine model. Am J Sports Med. 2009;37:160-7.
- [CrossRef] [PubMed] [Google Scholar]
- Cyclic pull-out strength of hamstring tendon graft fixation with soft tissue interference screws. Influence of screw length. Am J Sports Med. 1999;27:778-83.
- [CrossRef] [PubMed] [Google Scholar]
- Intraoperative complications using the Bio-transfix femoral fixation implant in anterior cruciate ligament reconstruction. Arch Orthop Trauma Surg. 2010;130:375-9.
- [CrossRef] [PubMed] [Google Scholar]
- Complications following hamstring anterior cruciate ligament reconstruction with femoral cross-pin fixation. Arthroscopy. 2005;21:1407-8.
- [CrossRef] [PubMed] [Google Scholar]
- Adjustable-versus fixed-loop devices for femoral fixation in ACL reconstruction: An in vitro full-construct biomechanical study of surgical technique-based tibial fixation and graft preparation. Orthop J Sports Med. 2018;6:2325967118768743.
- [CrossRef] [PubMed] [Google Scholar]
- Mechanical properties of suspensory fixation devices for anterior cruciate ligament reconstruction: Comparison of the fixed-length loop device versus the adjustable-length loop device. Knee. 2014;21:743-8.
- [CrossRef] [PubMed] [Google Scholar]
- Biomechanical comparisons of current suspensory fixation devices for anterior cruciate ligament reconstruction. Int Orthop. 2018;42:1291-6.
- [CrossRef] [PubMed] [Google Scholar]
- Three femoral fixation devices for anterior cruciate ligament reconstruction: Comparison of fixation on the lateral cortex versus the anterior cortex. Arthroscopy. 2010;26:796-807.
- [CrossRef] [PubMed] [Google Scholar]
- Adjustable buttons for ACL graft cortical fixation partially fail with cyclic loading and unloading. Knee Surg Sports Traumatol Arthrosc. 2019;27:2530-6.
- [CrossRef] [PubMed] [Google Scholar]
- A biomechanical comparison of femoral cortical suspension devices for soft tissue anterior cruciate ligament reconstruction under high loads. Am J Sports Med. 2015;43:154-60.
- [CrossRef] [PubMed] [Google Scholar]
- Suspensory fixation of grafts in anterior cruciate ligament reconstruction: A biomechanical comparison of 3 implants. Arthroscopy. 2009;25:767-76.
- [CrossRef] [PubMed] [Google Scholar]
- Fixed-versus adjustable-loop femoral cortical suspension devices for anterior cruciate ligament reconstruction: A systematic review and meta-analysis of biomechanical studies. Orthop J Sports Med. 2018;6:2325967118801762.
- [CrossRef] [PubMed] [Google Scholar]
- A comparative biomechanical study of femoral cortical suspension devices for soft-tissue anterior cruciate ligament reconstruction: Adjustable-length loop versus fixed-length loop. Arthroscopy. 2018;34:566-72.
- [CrossRef] [PubMed] [Google Scholar]
- Biomechanical evaluation of an adjustable loop suspensory anterior cruciate ligament reconstruction fixation device: The value of retensioning and knot tying. Arthroscopy. 2016;32:2050-9.
- [CrossRef] [PubMed] [Google Scholar]
- Biomechanical comparison of fixed-loop and adjustable-loop cortical suspensory devices for metaphyseal femoral-sided soft tissue graft fixation in anatomic anterior cruciate ligament reconstruction using a porcine model. Arthroscopy. 2017;33:1225-32.e1.
- [CrossRef] [PubMed] [Google Scholar]
- Adjustable loop ACL suspension devices demonstrate less reliability in terms of reproducibility and irreversible displacement. Knee Surg Sports Traumatol Arthrosc. 2018;26:1392-8.
- [CrossRef] [PubMed] [Google Scholar]
- Estimating the mean and variance from the median, range, and the size of a sample. BMC Med Res Methodol. 2005;5:13.
- [CrossRef] [PubMed] [Google Scholar]
- A biomechanical comparison of anterior cruciate ligament suspensory fixation devices in a porcine cadaver model. Clin Biomech (Bristol, Avon). 2014;29:230-4.
- [CrossRef] [PubMed] [Google Scholar]
- Assessing the risk of bias of individual studies in systematic reviews of health care interventions In: Methods Guide for Effectiveness and Comparative Effectiveness Reviews. Rockville, MD: Agency for Healthcare Research and Quality (US) AHRQ Methods for Effective Health Care; 2008.
- [Google Scholar]
- Quantifying heterogeneity in a meta-analysis. Stat Med. 2002;21:1539-58.
- [CrossRef] [PubMed] [Google Scholar]
- Measuring inconsistency in meta-analyses. BMJ. 2003;327:557-60.
- [CrossRef] [PubMed] [Google Scholar]
- Biomechanical evaluation of a novel loop retention mechanism for cortical graft fixation in ACL reconstruction. Orthop J Sports Med. 2020;8:2325967120904322.
- [CrossRef] [PubMed] [Google Scholar]
- Biomechanical testing of fixed and adjustable femoral cortical suspension devices for ACL reconstruction under high loads and extended cyclic loading. J Exp Orthop. 2020;7:27.
- [CrossRef] [PubMed] [Google Scholar]
- Systematic review of fixed-versus adjustable-loop femoral cortical suspension devices for anterior cruciate ligament reconstruction: Letter to the editor. Orthop J Sports Med. 2019;7:2325967119843504.
- [CrossRef] [PubMed] [Google Scholar]
- GraftLink® ACL reconstruction with the ACL tightrope® II fixation: Surgical technique.
- [Google Scholar]
- ToggleLoc™ and toggleloc XL for ACL reconstruction using a quadruple semitendinosus graft.
- [Google Scholar]
- Comparison of initial fixation strength between biodegradable and metallic interference screws and a press-fit fixation technique in a porcine model. Am J Sports Med. 1998;26:815-9.
- [CrossRef] [PubMed] [Google Scholar]
- A functional comparison of animal anterior cruciate ligament models to the human anterior cruciate ligament. Ann Biomed Eng. 1998;26:345-52.
- [CrossRef] [PubMed] [Google Scholar]
- Arthrometric aspects of anterior cruciate ligament surgery before and after reconstruction with patellar tendon grafts. Tech Orthop. 2005;20:421-38.
- [CrossRef] [Google Scholar]
- The fixation strength of six hamstring tendon graft fixation devices in anterior cruciate ligament reconstruction. Part I: Femoral site. Am J Sports Med. 2003;31:174-81.
- [CrossRef] [PubMed] [Google Scholar]
- Autografts commonly used in anterior cruciate ligament reconstruction. J Am Acad Orthop Surg. 2011;19:259-64.
- [CrossRef] [PubMed] [Google Scholar]
- Tendon healing in a bone tunnel differs at the tunnel entrance versus the tunnel exit: An effect of graft-tunnel motion? Am J Sports Med. 2006;34:1790-800.
- [CrossRef] [PubMed] [Google Scholar]
- Supplemental tibial fixation for anterior cruciate ligament reconstruction. Arthroscopy. 2008;24:1078-80.
- [CrossRef] [PubMed] [Google Scholar]
- Biomechanics of the anterior cruciate ligament and implications for surgical reconstruction. Strategies Trauma Limb Reconstr. 2007;2:1-12.
- [CrossRef] [PubMed] [Google Scholar]
- Fixed-versus adjustable-loop devices for femoral fixation in anterior cruciate ligament reconstruction: A systematic review. Arthroscopy. 2019;35:2484-98.
- [CrossRef] [PubMed] [Google Scholar]
- Adjustable loop femoral cortical suspension devices for anterior cruciate ligament reconstruction: A systematic review. Indian J Orthop. 2020;54:426-43.
- [CrossRef] [PubMed] [Google Scholar]