Translate this page into:
Recent advances and future trends in knee arthroscopy
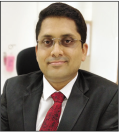
*Corresponding author: Nandan N. Rao, Department of Orthopaedics, Unity Hospital, Surat, Gujarat, India. drnandanortho@gmail.com
-
Received: ,
Accepted: ,
How to cite this article: Rao NN, Pardiwala DN. Recent advances and future trends in knee arthroscopy. J Arthrosc Surg Sport Med 2020;1(1):98-109.
Abstract
Knee arthroscopic surgery continues to advance in leaps and bounds. The anterior cruciate ligament (ACL) remains the most controversial structure to be operated on in the knee, and although the enthusiasm for double- bundle reconstruction has waned, there is a definite preference for anatomic reconstruction using an individualized approach. Despite early failed attempts at ACL primary repair, there is a renewed interest in this preservative approach with the application of technologically advanced instruments and implants. The thrust toward meniscal preservation too continues as better understanding of meniscal healing, improved repair techniques, and novel methods to enhance healing have encouraged surgeons to repair tears previously deemed irreparable. Similarly, articular cartilage surgery has advanced with better understanding of cellular mechanisms and the development of newer techniques of cartilage regeneration. Although the focus in the present era has been to restore anatomy with optimal biomechanical constructs, upcoming technology will address biological concerns in healing, especially with degenerate tissues. Future trends in knee arthroscopy include application of scaffolds, orthobiologics, stem cells, and bioreactors to generate personalized, and patient specific autogenous tissue engineered implants. This review article summarizes the latest developments in knee arthroscopy and predicts future trends in this rapidly advancing subspecialty.
Keywords
Knee ligament repair
Knee arthroscopy
Knee cartilage repair
Knee meniscus repair
Recent advances
INTRODUCTION
In recent years, there have been many advances in all orthopedic disciplines, and knee arthroscopy is no exception. These developments in knee arthroscopic surgery have been due to our better understanding of knee anatomy and biomechanics, and in turn, better management of ligament, meniscus, and articular cartilage injuries of the knee. These have been coupled with advances in optical technology, instrumentation, and implants which now enable precise, preservative, reconstructive, and regenerative surgery of the knee. As the science and application of arthroscopic knee surgery grows, we have noted a marked shift toward repairing intra-articular structures such as the menisci and anterior cruciate ligament (ACL) as a means to preserve knee anatomy. Ligaments and menisci not amenable to repair and healing are reconstructed, or transplanted, to restore anatomy. A host of innovative regenerative techniques and cell based technology is also now applied to treat articular cartilage lesions of the knee. This brief narrative describes the recent advances and future trends in knee arthroscopic surgery.
LIGAMENTS
ACL
ACL tears continue to be the most common, and controversial injury in orthopedic sports medicine. The latest trends in ACL surgery include ACL repair, individualized ACL reconstruction, remnant-sparing ACL reconstruction, the use of regenerative adjuncts to improve the biology of ACL graft ligamentization, and early stabilization in skeletally immature ACL deficient patients. There is also an increased recognition in the past few years of the roles of bone morphology, and hormones in the predisposition for ACL tears, with introduction of ACL prevention programs.
ACL repair
Anatomic ACL reconstruction has been able to give excellent results with a high rate of return to sports. However, there are lacunae in this reconstructive approach such as loss of proprioception, graft site morbidity, surgeon errors in tunnel placement, residual instability, and a prolonged process of graft revascularization and incorporation.[1] Hence, despite initial failure in this approach, there has been a resurgence of interest in ACL repair. Healing of the torn ACL following repair, provides an exciting opportunity of restoring knee stability with tissue which retains not only its native anatomy but also neurovascular supply.
The indications for ACL repair are acute femoral avulsions (Sherman Types I and II tears) of <3 weeks duration, with good quality tissue to be approximated, in young patients with lower activity levels and pre-injury Tegner activity scores <5. Isolated studies have documented good results in athletes and high activity groups too. However, the biggest hurdle to wide acceptability of ACL repair is the lack of convincing clinical data to support its regular use in clinical practice. This may change with time. At present, there are four different techniques of ACL repair described. These include refixation with suture anchors, internal brace ligament stabilization, dynamic intra-ligamentary stabilization (DIS), and bridge enhanced ACL repair (BEAR).
Refixation with suture anchors
Refixation with two suture anchors in the femoral footprint with alternate interlocking Bunnell type stitches in the two bundles of ACL has been described with varying success. Failure rates ranging from 15% to 25% have been reported.[2,3] Early results, especially in adolescent patients, have so far been encouraging.[4]
Internal brace ligament stabilization
Satisfactory 2-year patient outcomes and failure rates as low as 4.8% have been reported with internal brace ligament stabilization.[5] In this technique, the proximal ACL rupture is reattached to the lateral femoral condyle with a looped suture tied over a cortical button on the lateral femoral condyle. The same cortical button is also used to fix a non-absorbable braided suture passed through the femoral condyle, through the substance of the ACL and tibial footprint and fixed on the tibia with an anchor. The central non-absorbable suture tape gives mechanical protection to the healing ligament. The addition of the internal brace has been shown to reduce the failure rates from 13.8% (without internal brace) to 7.4% (with internal brace).[6] This technique has also been reported in three children aged 5–7 years, where the internal brace was removed at 3 months after healing. In all patients, the ACL healed on second look arthroscopy, and they returned to routine activities with results sustained at 2 years.[7] DIS This technique builds on the internal brace technique with the belief that traditional ACL repair fails due to cyclical loading of the repair. A polyethylene wire is fixed on the tibial side with a spring screw mechanism which holds the tibia in posterior translation during all degrees of movements of the knee. Thus, the repair is protected leading to enhanced healing. A systematic review of 15 studies with Levels 1–5 evidence suggests that ACL repair using this technique is a safe and effective procedure in a select group of patients. The failure rates vary from 4% to 13.6%, and the revision rates varied from 2.1% to 15% at 1 year, and 7.9–11% at 2 years. The study noted that the long-term failure rates are comparable to ACL reconstruction in properly selected patients.[8]
BEAR
The BEAR technique utilizes a bovine origin extracellular matrix scaffold augmentation in addition to the ACL repair to enhance healing of the torn ACL. The drawback is this technique requires a 5 cm arthrotomy. Only one human study has been done and reported good to excellent results at 2 years.[9]
Individualized ACL reconstruction
Although ACL repair shows some promise, the gold standard for ACL tears remains reconstructive surgery. What is increasingly recognized is that “one size does not fit all.” Anatomical variations in size and shape of the bones and ligaments, along with varied patient factors and functional demands may not allow the same standardized reconstruction in every knee. Hence, the concept of individualized ACL reconstruction has been proposed.[10] The principles of this include: Appreciate the native anatomy, individualize surgery according to patient needs, place the graft in the center of footprint, attempt to fill in about 80% of the footprint surface, and tension the grafts individually if performing a double-bundle construct. Routine notchplasty is discouraged as it may lead to bone overgrowth and impingement at a later date. Although the ideal graft, technique, fixation, and need for augmentation will continue to be debated, the following information, determined based on recent meta-analyses, may be applied to individualize ACL reconstruction.
Single-bundle versus double-bundle reconstruction
A Cochrane database review found no difference in pain, complications, graft failure, and subjective knee scores at short- and long-term follow-up in patients with single- and double-bundle ACL reconstruction. However, patients with double-bundle reconstruction were better in terms of IKDC knee examination, knee stability with KT-1000, rotational knee stability, with better prevention of meniscus injury.[11] A recent meta-analysis of randomized controlled trials with at least 5 year follow-up found no difference in the objective stability, subjective IKDC, Lysholm and Tegner scores, graft rupture, and osteoarthritis changes between a double- and single-bundle reconstruction. However, the meta-analysis included <300 patients from 5 studies. Hence, over the past 3 years, the enthusiasm for double-bundle reconstruction has waned.[12]
Graft choices in ACL reconstruction
No clear scientific data exist that clarifies whether one graft is superior to the other, however, there seems to be a slow shift in choice of graft, as hamstring tendons are now used at least as often as patellar tendon as an ACL graft because there appears to be less morbidity with hamstring graft use.
In a meta-analysis on graft choices for ACL reconstruction which compared objective stability scores, subjective IKDC and Lysholm scores, graft failure rates and pain scores, patients with a quadriceps tendon graft had lesser graft harvest site pain than BPTB graft, and better Lysholm score than hamstring autograft, while all the other variables were similar.[13] The rupture rates with BPTB grafts are lesser than hamstring autografts although the difference is miniscule. The objective stability and clinical outcomes do not differ.[14] Soft-tissue allografts are inferior to autografts for primary ACL reconstruction in terms of the lower IKDC score, and objective stability scores.[15] For revision knee reconstruction autografts and non-irradiated allografts have been found to have similar objective and subjective outcome scores. Irradiation of allografts makes them an inferior choice of graft for primary and revision cases.[16]
Graft comparative rate of infection
In terms of post-operative infection after ACL reconstruction, using a patellar tendon autograft has a 77% lesser incidence than hamstring autograft, and 66% lower incidence than all types of other grafts. At the same time, autografts and allografts did not differ in the incidence of infections.[17]
Antero-medial portal (AM) versus trans-tibial (TT) technique
A meta-analysis of randomized trials found that patients with AM technique had better subjective scores (IKDC, and Lysholm) and objective stability (Lachman’s, Pivot shift, and side to side difference) as compared to TT technique.[18]
Fixation devices
A Cochrane database review found very low quality evidence that bioabsorbable screws are associated with more treatment failures, including intra-operative breakage, although the subjective and objective outcome scores are similar.[19] A meta-analysis of level one studies did not find any difference between a cortical button, cross pin, and interference screw for fixation on the femoral side for hamstring autografts.[20] For quadrupled hamstring grafts aperture fixation led to >3 mm but <5 mm side to side difference on KT-1000 measurements and a significant increase in graft ruptures as compared to suspensory fixation. Other stability measures and patient outcome scores were similar.[21]
Return to sports
The rate of return to sports after a primary ACL reconstruction is 83%, with a 5.2% re-rupture rate.[22] The reported rates of return to sports at the same level after revision ACL reconstruction is only 43%, even if 73% patients had a good to excellent subjective and objective result.[23] In children and adolescents, the rate of return to pre-injury level of sport was 78.6% and that to competitive level of sport was 81.0%. Overall, 13% patients had graft re- ruptures, and in 14% contralateral ACL injuries were reported.[24]
Remnant sparing ACL reconstruction
With the recent trend of preservation and repair in knee arthroscopy, surgeons are increasingly sparing viable ACL remnants during reconstruction [Figure 1]. Evidence suggests that preservation of the ACL remnant is beneficial in terms of vascularity and proprioception, which may improve recovery of joint position sense, and enhances revascularization and integration of the graft.[25-27] Remnant sparing may be in the form of tibial remnant re-tensioning, specific bundle reconstruction for partial ACL tears, and remnant sparing where the graft is passed through the tibial tunnel and within the remnant. The most recent meta-analysis of Levels 1 and 2 studies determined that remnant sparing ACL reconstruction is not vastly superior to traditional ACL reconstruction, and there is no difference in the healing of grafts, or the overall complication rates. However, remnant sparing results in better objective stability in terms of KT 1000/2000 measurements, and better Lysholm scores.[28]

- Remnant sparing anterior cruciate ligament (ACL) reconstruction. (a) MRI reveals a recent ACL femoral avulsion of Sherman Type II (b) arthroscopic technique involves (i) identifying the ACL femoral avulsion and creating tunnels at the anatomical insertion sites, (ii) inserting a curved suture anchor anterior to the ACL femoral tunnel within the footprint of the ACL, (iii) passing one limb of the suture in mattress configuration through the ACL remnant, (iv) ACL graft passage while retracting the ACL stump, (v) a knot is now applied to the sutures so that the remnant envelopes the ACL graft and is fixed to the femoral insertion footprint.
Biologics in knee ligament surgery
Platelet-rich plasma (PRP), platelet-rich growth factor, and stem cells have all been used along with ACL reconstruction and repair in an attempt to improve graft healing. Despite encouraging results in pre-clinical studies, a systematic review and level one study concluded that the role of these is still controversial. There does not seem to be any improved graft maturation over time, and no benefits in clinical outcome, bone integration, and prevention of bone tunnel enlargement.[29] Autologous bone marrow aspirate concentrate (BMAC) has shown promise in ACL femoral avulsions without retraction. However, further level one studies would be needed to prove the utility of bone marrow procedures.[30]
ACL reconstruction in skeletally immature patients
Several Levels 3 and 4 studies, and a meta-analysis of the studies,[31] have proven that early surgery is preferable in skeletally immature patients as compared to non-operative treatment. Delaying treatment for more than 12 weeks results in four-fold rise in medial meniscus injuries, and increased odds ratio of lateral condyle and medial condyle chondral injuries of 5.6 and 11.3, respectively.[32] There are three techniques of ACL reconstruction in skeletally immature patients: Transphyseal, physeal-sparing, and hybrid techniques. It is recommended that whenever the physis needs to be violated the tunnel should be as perpendicular and central in the physis as possible. The technique to be chosen has been defined according to bone age and physeal growth potential.[33]
Role of bony morphology
An increased tibial slope, especially lateral tibial slope, has been shown to increase shear forces and stress across the ACL in biomechanical studies.[34] In a meta-analysis; an increased tibial slope has been associated with increased risk of ACL injury.[35] Decreasing posterior tibial slope has been shown to be vital in preventing failure in patients with increased tibial slope undergoing revision ACL reconstruction. It is believed that a posterior tibial slope of 13° or more needs to be corrected in the revision situation to around 5°.[36] The role of bony correction in primary ACL reconstruction is controversial. Other bone morphological features which are postulated to predispose to increased risk of ACL injury and failure of ACL reconstruction are shallow medial tibial plateau (deeper medial tibial plateau constrains the femur better), a shorter medial tibial plateau, lower volume of medial tibial spine, a dome shaped inter-condylar notch (pyramidal notch maintains better stability), and spherical medial femoral condyle (ovoid shape is more stable).[37] Further studies using 3D imaging and statistical shape modeling may help in the future to better delineate the role of bony morphological variations in knee stability. This may also help to predict athletes at high risk for ligament tears, and perhaps formulate better guidelines for bony correction in primary ACL reconstruction.
The effect of hormones and ACL injury prevention programs
In females, the relative risk of ACL tear is highest during the ovulatory phase, followed by the follicular phase, and least in the luteal phase. Oral contraceptive pills provided 20% reduction in risk of injury.[38] Women had higher rate of second ACL injuries (6.84%) than men (5.67%). Overall women suffer more re-ruptures and contra-lateral ACL tears as compared to men.[39]
A meta-analysis found that ACL prevention programs did decrease the incidence of ACL injuries in all athletes by 50%, especially in female athletes.[40] Neuromuscular and proprioceptive training also decreases the rate of injuries, but no specific group of exercises is superior.[41]
Antero-lateral ligament (ALL) and lateral extra-articular tenodesis (LET)
The persistence of rotatory instability after ACL reconstruction has renewed interest in the anterolateral extra-articular stabilizing structures called the ALL. The anatomy, imaging features, role in knee stability, indications, and technique of ALL reconstruction have been debated.[42] The ALL expert group has published a consensus that ALL reconstruction affords internal rotation stability and reduced pivot shift after an ACL reconstruction.[43] Anatomic ALL reconstruction, in addition to ACL reconstruction, has been advocated in patients at high risk of ACL re-rupture, such as age <20 years, athletes engaged in pivoting sports, and patients with generalized ligament laxity. In patients with a Grade 3 pivot shift undergoing revision ACL reconstruction, an anatomic ALL reconstruction may be done to reduce the chances of failure. An alternative to ALL reconstruction is a LET which essentially involves a central band of iliotibial band being re-routed under the LCL and fixed to the tibia.[42-44] A level one study found that the addition of an extra-articular procedure decreases the pivot shift, and improves stability of the ACL reconstruction, while the subjective and objective scores remain the same.[45]
Posterior cruciate ligament (PCL)
The PCL remains less controversial as compared to the ACL and there has been increased consensus in its surgical treatment. There are various approaches described for PCL reconstruction, including single- or double-bundle, TT, and tibial inlay technique. A meta-analysis has demonstrated that although double-bundle reconstruction gives better objective posterior tibial stability and IKDC scores, patient reported outcomes are similar, and no technique is superior.[46] This added stability has been postulated to be important in the long term, and hence double-bundle PCL reconstruction is preferable. Similarly, both TT and tibial inlay methods are equivalent in terms of patient reported outcomes and objective scores with the tibial inlay technique being more prone to complications.[47] Dynamic bracing has been advised in the early phases of rehabilitation after PCL surgery. This brace offloads the PCL during deeper knee flexion angles and improves outcomes.[48] A meta-analysis found no difference in clinical outcomes between a single-bundle autograft and allograft PCL reconstruction. The patients with allograft had a longer duration of fever, higher white blood cell count, with a lower Tegner activity score, and lower objective stability scores.[49,50] Much like ACL, interest has been rekindled in PCL repair and recent studies reinforce the repair with suture tape. Long-term results and further developments in this field are awaited.[51]
Multiple ligament knee injury and knee dislocations
Knee dislocation presents a unique challenge in terms of the management of soft-tissue envelope, repair, and/or reconstruction of ligaments, with their timing and technique. Although an individualized approach is recommended for these complex injuries, numerous studies have enabled a consensus and algorithm for management of these challenging injuries.[52,53]
MENISCUS
The importance of the meniscus for normal knee function has been greatly appreciated in the past decade. It is increasingly recognized that it is important to repair, replace, or regenerate injured meniscus to prevent the progression of osteoarthritis. Meniscal preservation using different techniques has been shown to improve the clinical outcomes and delay degeneration. Meniscal repair can be achieved by all-inside, outside in, and inside out techniques and a variety of devices and instruments are available for the same [Figure 2].

- Meniscus repair for complex tear. (a) and (b) MRI reveals a complex displaced transection of lateral meniscus with locked knee. (c) Arthroscopic technique involves (i) reduction of the locked displaced meniscus fragment, (ii) identifying the complex tear pattern to involve a peripheral longitudinal component and a radial component, (iii) suturing each component of the tear with an all-inside technique, (iv) so as to achieve anatomical restoration of the lateral meniscus, (v) second-look arthroscopy after 4 months reveals a completely healed meniscus tear.
Augmentation of meniscus repairs
Even with the latest techniques of meniscus repair failure rates as high as 24% have been reported, and are related to various factors including tear pattern, vascular anatomy, age, and limb alignment. Simple augmentation techniques including trephination, rasping, and abrasion of the synovium have been shown to improve healing in repaired meniscal tears.[54] Techniques such as fibrin clots sutured at the meniscus repair site, and PRP used either alone or with a variety of scaffolds, have given equivocal evidence in clinical studies. Further high level studies are necessary to prove their usefulness.[54] Newer methods which may hold promise are arthroscopic collagen sheath wrapping of complex tears with bone marrow aspirate injected under it, and a chitosan- PRP composite implant.[54]
Meniscal substitution
In some instances meniscus repair may not be feasible, and patients may have segmental, subtotal, or total loss of menisci. In such situations, meniscal substitution in the form of allograft transplant for subtotal or total meniscal loss, and artificial meniscal scaffolds for segmental defects are a viable option. In all these cases, the patient should be between 16 and 50 years of age, with a BMI <35, the knee should be stable with a normal alignment, and the cartilage should be well preserved with a ICRS grade of 2 or less.
Meniscal allografts
These have consistently given good results in appropriately selected patients in the long term.[55] However, there are concerns which include graft size mismatch, questionable long-term chondroprotection, low rate of return to sports, progressive joint degeneration, graft sterility and incompatibility, limited availability in many countries, and low rates of remodeling and stability.
Meniscal scaffolds
It may be useful when there is segmental loss of meniscus <5 cm with an intact peripheral rim. A scaffold theoretically allows for native cells and tissue to grow in, while it biodegrades over a period of time. There are two different types of scaffolds: Collagen meniscus implant (CMI) made from bovine collagen matrix, and polycaprolactone- polyurethane scaffold (Actifit). Both have shown mixed results in patients with up to 10 year follow-up for CMI device,[56] and up to 6 years follow-up in case of Actifit device.[57] In a systematic review, both the devices have been found to be similar in terms of patient outcomes.[58] A 12.6% rate of complications has been reported which includes pain, effusion, infections, debridement for non-integrated scaffolds, re-tears, and scar tissue removal. A failure rate of 9.9% at 40 months for Actifit, and 6.7% at 44 months, for CMI device have been reported.[58] A scaffold which can produce high quality in vivo results in everyone has not yet been found.
Tissue engineering and regenerative medicine for scaffolds
Research is progressing in this field whereby these scaffolds will be 3D printed and impregnated with either autologous cells from the meniscus, or stem cells, and growth factors, which can then be cultivated in a bioreactor to get a patient-specific tissue engineered meniscal implant which is fully biocompatible.[59]
Gene therapy for meniscus regeneration
Encouraging results have been reported by gene therapy, whereby favorable genes can be introduced into target meniscal cells to encourage regeneration.[60]
CARTILAGE
Cartilage defects and injuries are a formidable challenge in regenerative medicine. Cartilage treatment strategies can be characterized as palliation (chondroplasty and debridement), repair (drilling and microfracture), or restoration (autologous chondrocyte implantation [ACI], osteochondral autograft transfer [OAT], and osteochondral allograft [OCA]).
OATS and ACI
While OAT does result in hyaline cartilage regeneration; it has its own disadvantages, most important being its inability to be used in lesions larger than 3 cm2. ACI has shown excellent results with all three generations [Figure 3]. The present generation of matrix ACI and fibrin ACI has the advantage of three-dimensional culture of chondrocytes which decreases the chondrocyte dedifferentiation noticed with earlier generations of the technique.[61] The key disadvantage of ACI remains that it requires a two stage procedure, and newer generations of this technique will aim toward a one-stage allograft ACI.

- (a) Large osteochondritis dissecans (OCD) of medial femoral condyle of knee, (b) fibrin autologous chondrocyte implantation (ACI) cartilage repair, (c) and (d) 2-year follow-up MRI reveals a successful cartilage repair with MOCART score of 95, (e) T2 cartilage map performed 2 years after implantation reveals a hyaline rich cartilage repair (white arrows denote the cartilage repair site in c,d,e).
Scaffolds
Scaffold based cartilage regeneration can be applied for chondral or osteochondral defects. For chondral defects the defect may be repaired in one or two stages. The two stage technique utilizes the cartilage cells obtained from a normal area of the knee in the first stage, cultured in vitro, and impregnated into a variety of scaffolds. The scaffolds could be porcine collagen matrix, or a variety of synthetic substrates such as polylactic acid and polyglycolic acid, or natural substrates such as hyaluronic acid, collagen derivatives, agarose, alginate, or fibrin glue.[62] Single stage procedures utilize scaffolds impregnated with either fragmented cartilage tissue obtained from a healthy area of the knee or bone marrow. Another approach called as autologous matrix induced chondrogenesis (AMIC) utilizes a porcine collagen matrix to stabilize a blood clot obtained from microfracture. “AMIC plus” adds PRP for further biologic stimulation.
For osteochondral defects, scaffolds have been developed with a two or three layered structure, and are currently under investigation.[62] Similarly, 3D printed personalized scaffolds, nanofiber technology, and hydrogels are being investigated, and would be incorporated in cartilage repair in the future. 4D scaffolds are also an interesting development. These grafts are made from biomaterials which will respond to external stimuli and modify accordingly.[63]
Orthobiologics in focal cartilage defects
The orthobiologics used in cartilage defects are amniotic membrane derived mesenchymal stem cells (MSC), adipose- derived MSC, BMAC, PRP, and growth factors (BMP4 and BMP7).
MSCs either used alone or with scaffolds have shown promise in animal models but have not shown significant benefits in humans. Intra-articular injections of MSC have not been shown to improve outcomes in osteoarthritis.[64]
BMAC contains a large number of growth factors and cytokines which help in cartilage regeneration and healing. It has been used as an adjunct to microfracture alone, microfracture with BMAC and HA, and microfracture supplemented with a scaffold impregnated with BMAC. Human studies with scaffolds have shown complete defect filling in 80–100% of patients.[65] Clinical improvement has been reported in up to 100% patients at 5 years, and on second look arthroscopy was found to have regenerated near normal hyaline cartilage.[66] BMAC also been reported to give better results in OCA transplants in terms of graft incorporation and healing when used as an adjunct.[67]
PRP has shown good results in the treatment of osteoarthritis, but the results are mixed in focal chondral defects. This may be due the non-standardized ways of its preparation and heterogeneous reporting standards. It has been used in conjunction with micronized allograft articular cartilage, fibrin glue, scaffolds, and demineralized bone matrix for osteochondral defects; all with mixed results.[65]
BMP-4 and BMP-7 are the two main growth factors which are tested in animal models and have shown good cartilage growth and hyaline cartilage differentiation.[65]
The role of limb realignment including high tibial osteotomy as an adjunct to cartilage repair is important. The rationale is changing the mechanical axis of the lower limb so as to transfer the body weight across to healthy articular cartilage. Several studies have shown that accurate correction is the leading predictor for success.[68,69]
PATELLOFEMORAL JOINT
Management of patellofemoral joint pathology is challenging as a result of the unique and complex organization of static forces and dynamic factors contributing to its functional capacity. The evaluation and treatment of patellofemoral instability (PFI) is based on static evaluation of the joint. Dynamic evaluation of this pathology may help us give better results. Various developments in this field in the form of cine MRI, dynamic ultrafast MRI, diffuse tension MRI, and its combination with fluoroscopy and ultrasound have given deeper insights into this pathology. Based on these, PFI measurements such as a quadriceps active ratio[70] and modified lateral patellar edge measurement have been proposed.[71] Recently, wearable devices have also been developed for dynamic evaluation of PFI. In future, Level 1 studies need to be developed to test these new diagnostic methods for better development of treatment methods.
The role of the medial patellofemoral ligament (MPFL) and its anatomy have been investigated for many years. Recent studies have defined a more broader medial attachment of the MPFL fanning across the upper half of the patella and the distal medial quadriceps tendon. Hence, this structure has now been called the medial patello-femoral complex. There is considerable variability in this attachment with 57.3% of this complex attached to the patella and the rest attached to the quadriceps tendon.[72] In the skeletally immature patients the femoral origin of the MPFL has a wide variability, with the average origin 4.7–10 mm distal to the femoral physis with the superior most fibers overlying the physis.[73] Fulkerson defined a new entity called medial quadriceps tendon femoral ligament, its attachments, role in lateral patellar stability, and its reconstruction technique. The medial attachment is to the distal quadriceps tendon, and femoral attachment is distal and anterior to the adductor tubercle immediately above the MPFL attachment.[74]
Although the position of the knee during MPFL tensioning is varied; biomechanical studies confirm that a 2N tension with the knee placed in 30–60° of flexion is the best.[75] Practically, tensioning such that a lateral patellar translation of one quadrant is achieved, at 30° knee flexion, is the best way to ensure avoiding over constraining the patella.[76,77] A double-bundle graft has been shown to decrease the graft failure rates from between 10.6%-26.9% in single bundle grafts to 4.5%-5.5% in double bundle grafts.[78,79] Several new techniques have been described to avoid the complications associated with patella tunnels and suture anchors. One such technique utilizes the central quadriceps tendon 10–12 mm in width and 3 mm depth left attached to the patella and rerouted in layer two of the knee to be fixed in the femoral isometric tunnel with an interference screw.[77]
MPFL repair may be indicated in young patients having first time dislocation with a patellar osteochondral fragment which needs fixation, where a distinct rupture from the patella, or less commonly from the femur, can be recognized. Similarly, imbrication of the MPFL may be sufficient in knees where bony realignment procedures have resulted in stable patella in the trochlea with a lax medial retinacular structure.[80]
Patellofemoral cartilage defects
These present unique challenges as patella-femoral joint loads may reach 6.5 times body weight, patella has the thickest cartilage in the body, femoral cartilage may not a good substitute, and an arthrotomy is needed to manage patellar cartilage defects.[81] For this reason, microfracture and bone marrow stimulation techniques have not been able to replicate the good results seen in the tibiofemoral joint. For smaller lesions (<3 cm2) OAT, and for larger lesions (>2 cm2) ACI and OCA have given consistently good results with lower rates of failure seen in ACI (5% vs. 16%). Hence, it is suggested that OCA be reserved for salvage and revision situations.[82] The medial and lateral trochlea which are the usual donors for OATS can be used in these cases for only small patellar defects. Scaffolds for patellar cartilage regeneration have given disappointing results with a 90% failure and 70% re-operation rate. Going forward scaffolds with better stress resistance need to be developed.[82]
Algorithmic approach to recurrent lateral patellar instability
The goals of patellofemoral realignment surgery should be to create both a stable environment for optimal extensor mechanism performance, and an appropriate load transmission for optimal cartilage wear and joint loading. An algorithmic approach has been proposed to deal with all aspects of lateral patellar instability.[83]
For genu valgum more than 10° with more than 1 year of growth remaining a medial femoral epiphysiodesis is necessary. Similarly, if there is excessive femoral or tibial valgus after skeletal maturity then a medial closing wedge osteotomy is necessary
Excessive femoral anteversion >20° and external tibial extorsion should be corrected by derotational osteotomy. Femoral derotation may be performed at the subtrochanteric level or at the distal metaphysis. Tibial torsion is corrected at proximal physis
If alignment is acceptable, the tibial tubercle to trochlear groove distance beyond 20 mm, calculated preferably on CT scan, or less so on MRI, should be corrected to between 10 and 15 mm with an anteromedialization of the tibial tubercle (Fulkerson). Patella alta can be calculated on MRI using the patella-trochlear index or Caton-Deschamps index, which if more than 1.3 should be corrected with distalization of the tibial tubercle
Trochlear dysplasia has been classified into four types. Type A usually does not require correction and only an MPFL reconstruction is sufficient. Types B and D can be treated with sulcus deepening trochleoplasty [Figure 4]. Type C warrants a complex procedure with lack of evidence, and includes lateral facet elevation, proximal recession wedge trochleoplasty, or groove deepening trochleoplasty
MPFL tear requires a MPFL reconstruction as detailed earlier.
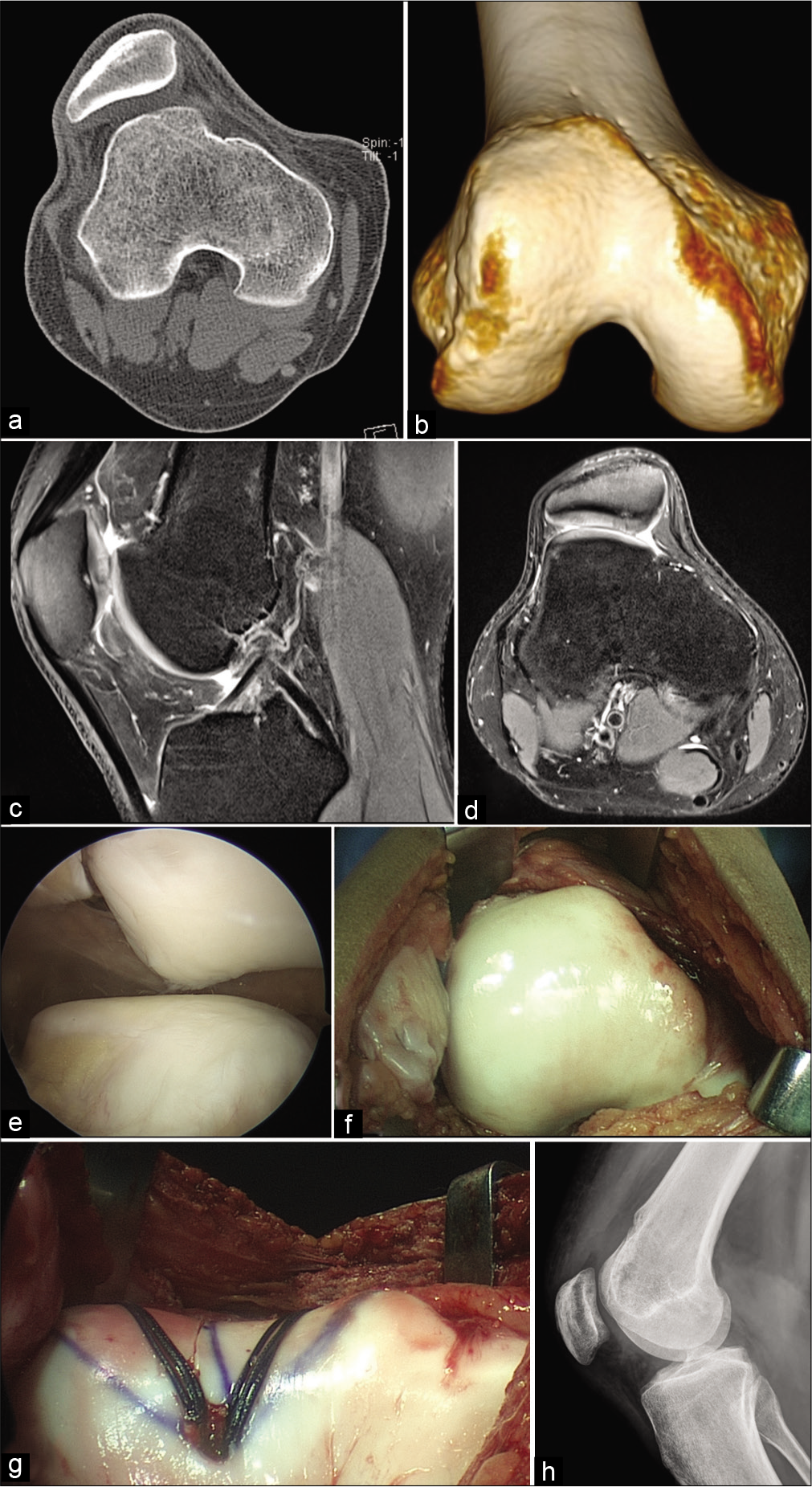
- Recurrent patellar lateral dislocations with severe trochlear dysplasia and MPFL tear. (a) CT scan axial cut reveals a Dejour Type 4 trochlear dysplasia, (b) 3D CT scan further defines the bony deformity, (c) MRI sagittal cut reveals the supratrochlear spur, (d) MRI axial cut demonstrates patellar chondral status, (e) arthroscopic evaluation from superolateral portal reveals lateral patellar mal-tracking with trochlear dysplasia and minimal patellar chondral lesion, (f) an open exposure for trochleoplasty is performed, (g) groove deepening trochleoplasty is completed with lateralization of the trochlear groove to compensate for increased tibial tubercle to trochlear groove distance, (h) post- operative lateral radiograph at 3 months reveals healing of trochleoplasty with anatomical femoral insertion site for MPFL reconstruction.
COMPUTER-ASSISTED NAVIGATION IN KNEE ARTHROSCOPIC SURGERY (CANS OR CAS)
Computer-assisted navigation was developed to improve the accuracy of tunnel positioning in knee ligament reconstructions, and enable precise correction in osteotomy around the knee. While there are conflicting reports on its utility, many studies support the use of navigation. The added invasiveness and complexity, along with huge increase in costs, have limited the use of CANS in routine knee arthroscopic ligament surgery.[84]
At present, it is used to study knee kinematics and in the following research applications:
To compare the efficacy of different techniques of ACL reconstruction
To assess the efficacy and need for extra-articular procedures
Scientific tool in studying the pivot shift test
Pre- and post-operative outcomes
For individualizing ACL reconstruction and in difficult revision situations.
ROBOTIC AND IMAGE GUIDED KNEE ARTHROSCOPY
Knee arthroscopy is technically demanding. Current arthroscopes do not provide depth of vision, are rigid, cannot negotiate curved spaces in the knee easily, and have a limited field of vision. All these factors, along with human error, can lead to suboptimal outcomes. While all the advancements given below are in the development stage, advances in robotics can help in the future.[85] There are presently four areas of development: Steerable robotic tools, leg manipulators, miniature stereo cameras, and 3D/4D imaging systems.
Steerable robotic tools have extra degrees of freedom, such as bending and rotation, which help to reach and perform the intended procedure with ease. These will have sensors which will make it easy to detect, and steer the path, to the desired location, and plan the surgery.
Autonomous leg manipulators will have algorithms fed into the system depending on the surgeon preference, type of surgery, and stage of surgery. This along with sensors placed outside the knee, and in the arthroscope, will allow the leg holder to position the knee accurately.
Robotic tools need depth realization to work in space. Hence, miniature stereoscopic cameras are being developed to gain depth information. Along with 3D/4D ultrasound modalities being developed, they will help in mapping the anatomy of the soft-tissue inside and outside the knee such as the ligaments, menisci, neurovascular structures, and tendons to allow for surgery.
An extension of the robotic technology may in the future allow for full autonomous surgery under the supervision of an expert.
CONCLUSION
Recent advances in arthroscopic knee surgery have enabled accurate restoration of anatomy with successful techniques involving repair, reconstruction, and replacement. With research and studies progressing in multiple directions, better outcomes in biological repair and augmentation can be expected in the future. Rapid strides in tissue engineering, regenerative medicine, and understanding of cellular mechanism, may help in the future to obviate the need for harvesting autografts and allografts. Technology may offer individualized human tissue scaffolds to replace ligaments, cartilage, and menisci, if repair at an early stage fails.
Declaration of patient consent
Patients consent not required as patients identity is not disclosed or compromised.
Financial support and sponsorship
Nil.
Conflicts of interest
Dr. Dinshaw N. Pardiwala is on the Editorial Board of the Journal.
References
- Arthroscopic primary repair of the anterior cruciate ligament: A narrative review of the current literature. JSM Sports Med Res. 2019;3:1-10.
- [Google Scholar]
- Arthroscopic primary repair of proximal anterior cruciate ligament tears. Arthrosc Tech. 2016;5:e1057-61.
- [CrossRef] [PubMed] [Google Scholar]
- Primary single suture anchor re-fixation of anterior cruciate ligament proximal avulsion tears leads to good functional mid-term results: A preliminary study in 12 patients. J Orthop Surg Res. 2017;12:171.
- [CrossRef] [PubMed] [Google Scholar]
- Arthroscopic anterior cruciate ligament repair for proximal anterior cruciate ligament tears in skeletally immature patients: Surgical technique and preliminary results. Knee. 2017;24:40-8.
- [CrossRef] [PubMed] [Google Scholar]
- Anterior cruciate ligament repair with independent suture tape reinforcement: A case series with 2-year follow-up. Knee Surg Sports Traumatol Arthrosc. 2019;27:60-7.
- [CrossRef] [PubMed] [Google Scholar]
- Arthroscopic primary repair of proximal anterior cruciate ligament tears: Outcomes of the first 56 consecutive patients and the role of additional internal bracing. Knee Surg Sports Traumatol Arthrosc. 2019;27:21-8.
- [CrossRef] [PubMed] [Google Scholar]
- Paediatric ACL repair reinforced with temporary internal bracing. Knee Surg Sports Traumatol Arthrosc. 2016;24:1845-51.
- [CrossRef] [PubMed] [Google Scholar]
- Dynamic intraligamentary stabilization for ACL repair: A systematic review. Knee Surg Sports Traumatol Arthrosc. 2019;27:13-20.
- [CrossRef] [PubMed] [Google Scholar]
- Bridge-enhanced anterior cruciate ligament repair: Two-year results of a first-in-human study. Orthop J Sports Med. 2019;7:2325967118824356.
- [CrossRef] [PubMed] [Google Scholar]
- Anatomic anterior cruciate ligament reconstruction utilizing the double-bundle technique. J Orthop Sports Phys Ther. 2012;42:184-95.
- [CrossRef] [PubMed] [Google Scholar]
- Double-bundle versus single-bundle reconstruction for anterior cruciate ligament rupture in adults. Cochrane Database Syst Rev. 2012;11:CD008413.
- [CrossRef] [PubMed] [Google Scholar]
- Single-bundle versus double-bundle autologous anterior cruciate ligament reconstruction: A meta-analysis of randomized controlled trials at 5-year minimum follow-up. J Orthop Surg Res. 2018;13:50.
- [CrossRef] [PubMed] [Google Scholar]
- Anterior cruciate ligament reconstruction: A systematic review and meta-analysis of outcomes for quadriceps tendon autograft versus bone-patellar tendon-bone and hamstring-tendon autografts. Am J Sports Med. 2019;47:3531-40.
- [CrossRef] [PubMed] [Google Scholar]
- Hamstring autograft versus patellar tendon autograft for ACL reconstruction: Is there a difference in graft failure rate? A meta-analysis of 47,613 patients. Clin Orthop Relat Res. 2017;475:2459-68.
- [CrossRef] [PubMed] [Google Scholar]
- Comparison of clinical outcomes after anterior cruciate ligament reconstruction with hamstring tendon autograft versus soft-tissue allograft: A meta-analysis of randomised controlled trials. Int J Surg. 2018;56:174-83.
- [CrossRef] [PubMed] [Google Scholar]
- Does the type of graft affect the outcome of revision anterior cruciate ligament reconstruction? A meta-analysis of 32 studies. Bone Joint J. 2017;99B:714-23.
- [CrossRef] [PubMed] [Google Scholar]
- Meta-analysis of the risk of infections after anterior cruciate ligament reconstruction by graft type. Am J Sports Med. 2018;46:1500-8.
- [CrossRef] [PubMed] [Google Scholar]
- Anteromedial versus transtibial technique in single-bundle autologous hamstring ACL reconstruction: A meta-analysis of prospective randomized controlled trials. J Orthop Surg Res. 2017;12:167.
- [CrossRef] [PubMed] [Google Scholar]
- Bioabsorbable versus metallic interference screws for graft fixation in anterior cruciate ligament reconstruction. Cochrane Database Syst Rev. 2016;7:CD009772.
- [CrossRef] [PubMed] [Google Scholar]
- No difference in outcomes between femoral fixation methods with hamstring autograft in anterior cruciate ligament reconstruction-a network meta-analysis. Knee. 2019;26:292-301.
- [CrossRef] [PubMed] [Google Scholar]
- Suspensory versus aperture fixation of a quadrupled hamstring tendon autograft in anterior cruciate ligament reconstruction: A meta-analysis. Am J Sports Med. 2017;45:2418-27.
- [CrossRef] [PubMed] [Google Scholar]
- Eighty-three per cent of elite athletes return to preinjury sport after anterior cruciate ligament reconstruction: A systematic review with meta-analysis of return to sport rates, graft rupture rates and performance outcomes. Br J Sports Med. 2018;52:128-38.
- [CrossRef] [PubMed] [Google Scholar]
- Revision anterior cruciate ligament reconstruction: Clinical outcome and evidence for return to sport. Knee Surg Sports Traumatol Arthrosc. 2015;23:2825-45.
- [CrossRef] [PubMed] [Google Scholar]
- Over 90 % of children and adolescents return to sport after anterior cruciate ligament reconstruction: A systematic review and meta-analysis. Knee Surg Sports Traumatol Arthrosc. 2018;26:1019-36.
- [CrossRef] [PubMed] [Google Scholar]
- Acceleration of tendon-bone healing of anterior cruciate ligament graft using autologous ruptured tissue. Am J Sports Med. 2012;40:1296-302.
- [CrossRef] [PubMed] [Google Scholar]
- Therapeutic advantage in selective ligament augmentation for partial tears of the anterior cruciate ligament: Results in an animal model. Am J Sports Med. 2013;41:365-73.
- [CrossRef] [PubMed] [Google Scholar]
- ACL reconstruction preserving the ACL remnant achieves good clinical outcomes and can reduce subsequent graft rupture. Orthop J Sports Med. 2013;1:2325967113505076.
- [CrossRef] [PubMed] [Google Scholar]
- Is remnant preservation in anterior cruciate ligament reconstruction superior to the standard technique? A systematic review and meta-analysis. Biomed Res Int. 2019;2019:1652901.
- [CrossRef] [PubMed] [Google Scholar]
- Has platelet-rich plasma any role in anterior cruciate ligament allograft healing? Arthroscopy. 2009;25:1206-13.
- [CrossRef] [PubMed] [Google Scholar]
- Anterior cruciate ligament tears treated with percutaneous injection of autologous bone marrow nucleated cells: A case series. J Pain Res. 2015;8:437-47.
- [CrossRef] [PubMed] [Google Scholar]
- Anterior cruciate ligament tears in children and adolescents: A meta-analysis of nonoperative versus operative treatment. Am J Sports Med. 2014;42:2769-76.
- [CrossRef] [PubMed] [Google Scholar]
- Degeneration of the knee joint in skeletally immature patients with a diagnosis of an anterior cruciate ligament tear: Is there harm in delay of treatment? Am J Sports Med. 2011;39:2582-7.
- [CrossRef] [PubMed] [Google Scholar]
- Management of the anterior cruciate ligament-injured knee in the skeletally immature athlete. Clin Sports Med. 2017;36:35-52.
- [CrossRef] [PubMed] [Google Scholar]
- Quantification of the role of tibial posterior slope in knee joint mechanics and ACL force in simulated gait. J Biomech. 2015;48:1899-905.
- [CrossRef] [PubMed] [Google Scholar]
- The influence of the tibial plateau slopes on injury of the anterior cruciate ligament: A meta-analysis. Knee Surg Sports Traumatol Arthrosc. 2014;22:53-65.
- [CrossRef] [PubMed] [Google Scholar]
- Tibial slope correction combined with second revision ACL produces good knee stability and prevents graft rupture. Knee Surg Sports Traumatol Arthrosc. 2015;23:2846-52.
- [CrossRef] [PubMed] [Google Scholar]
- The influence of tibial and femoral bone morphology on knee kinematics in the anterior cruciate ligament injured knee. Clin Sports Med. 2018;37:127-36.
- [CrossRef] [PubMed] [Google Scholar]
- The effect of menstrual cycle and contraceptives on ACL injuries and laxity: A systematic review and meta-analysis. Orthop J Sports Med. 2017;5:2325967117718781.
- [CrossRef] [PubMed] [Google Scholar]
- Influence of patient demographics and graft types on ACL second injury rates in ipsilateral versus contralateral knees: A systematic review and meta-analysis. Int J Sports Phys Ther. 2018;13:561-74.
- [CrossRef] [PubMed] [Google Scholar]
- Meta-analysis of meta-analyses of anterior cruciate ligament injury reduction training programs. J Orthop Res. 2018;36:2696-708.
- [CrossRef] [PubMed] [Google Scholar]
- Prevention of knee and anterior cruciate ligament injuries through the use of neuromuscular and proprioceptive training: An evidence-based review. J Athl Train. 2017;52:1171-2.
- [CrossRef] [PubMed] [Google Scholar]
- The anterolateral complex and anterolateral ligament of the knee. J Am Acad Orthop Surg. 2018;26:261-7.
- [CrossRef] [PubMed] [Google Scholar]
- Anterolateral ligament expert group consensus paper on the management of internal rotation and instability of the anterior cruciate ligament-deficient knee. J Orthop Traumatol. 2017;18:91-106.
- [CrossRef] [PubMed] [Google Scholar]
- Current concepts of the anterolateral ligament of the knee: Anatomy, biomechanics, and reconstruction. Am J Sports Med. 2018;46:1235-42.
- [CrossRef] [PubMed] [Google Scholar]
- Does combined intra-and extraarticular ACL reconstruction improve function and stability? A meta-analysis. Clin Orthop Relat Res. 2015;473:2609-18.
- [CrossRef] [PubMed] [Google Scholar]
- Single-bundle and double-bundle posterior cruciate ligament reconstructions: A systematic review and meta-analysis of 441 patients at a minimum 2 years' follow-up. Arthroscopy. 2017;33:2066-80.
- [CrossRef] [Google Scholar]
- Single-bundle versus double-bundle posterior cruciate ligament reconstruction: A meta-analysis of randomized controlled trials. Knee Surg Relat Res. 2017;29:246-55.
- [CrossRef] [PubMed] [Google Scholar]
- Quantification of functional brace forces for posterior cruciate ligament injuries on the knee joint: An in vivo investigation. Knee Surg Sports Traumatol Arthrosc. 2015;23:3070-6.
- [CrossRef] [PubMed] [Google Scholar]
- Comparison of autograft and allograft tendons in posterior cruciate ligament reconstruction: A meta-analysis. Medicine (Baltimore). 2017;96:e7434.
- [CrossRef] [PubMed] [Google Scholar]
- Graft sources do not affect to the outcome of transtibial posterior cruciate ligament reconstruction: A systematic review. Arch Orthop Trauma Surg. 2018;138:1103-16.
- [CrossRef] [PubMed] [Google Scholar]
- Posterior cruciate ligament repair with suture tape augmentation. Arthrosc Tech. 2018;8:e7-10.
- [CrossRef] [PubMed] [Google Scholar]
- Knee dislocations in sports injuries. Indian J Orthop. 2017;51:552-62.
- [CrossRef] [PubMed] [Google Scholar]
- Knee dislocations: Classification and treatment algorithm. In: Margheritini F, Espregueira-Mendes J, Gobbi A, eds. Complex Knee Ligament Injuries. Berlin, Heidelberg: Springer; 2019.
- [CrossRef] [Google Scholar]
- Augmentation techniques for meniscus repair. J Knee Surg. 2018;31:99-116.
- [CrossRef] [PubMed] [Google Scholar]
- Twenty-six years of meniscal allograft transplantation: Is it still experimental? A meta-analysis of 44 trials. Knee Surg Sports Traumatol Arthrosc. 2011;19:147-57.
- [CrossRef] [PubMed] [Google Scholar]
- Outcome after partial medial meniscus substitution with the collagen meniscal implant at a minimum of 10 years' follow-up. Arthroscopy. 2011;27:933-43.
- [CrossRef] [PubMed] [Google Scholar]
- Polyurethane-based cell-free scaffold for the treatment of painful partial meniscus loss. Knee Surg Sports Traumatol Arthrosc. 2017;25:459-67.
- [CrossRef] [PubMed] [Google Scholar]
- Similar clinical outcomes following collagen or polyurethane meniscal scaffold implantation: A systematic review. Knee Surg Sports Traumatol Arthrosc. 2018;26:2259-69.
- [CrossRef] [PubMed] [Google Scholar]
- Treatments of meniscus lesions of the knee: Current concepts and future perspectives. Regen Eng Transl Med. 2017;3:1-19.
- [CrossRef] [Google Scholar]
- Advanced regenerative strategies for human knee meniscus. In: Regenerative Strategies for the Treatment of Knee Joint Disabilities. New York: Springer; 2017. p. :271-85.
- [CrossRef] [Google Scholar]
- Advances and prospects in stem cells for cartilage regeneration. Stem Cells Int. 2017;2017:4130607.
- [CrossRef] [PubMed] [Google Scholar]
- Scaffold-based repair for cartilage healing: A systematic review and technical note. Arthroscopy. 2013;29:174-86.
- [CrossRef] [PubMed] [Google Scholar]
- Advancements and frontiers in nano-based 3D and 4D scaffolds for bone and cartilage tissue engineering. Int J Nanomedicine. 2019;14:4333-51.
- [CrossRef] [PubMed] [Google Scholar]
- Intra-articular injection of mesenchymal stem cells for clinical outcomes and cartilage repair in osteoarthritis of the knee: A meta-analysis of randomized controlled trials. Arch Orthop Trauma Surg. 2019;139:971-80.
- [CrossRef] [PubMed] [Google Scholar]
- Orthobiologics for focal articular cartilage defects. Clin Sports Med. 2019;38:109-22.
- [CrossRef] [PubMed] [Google Scholar]
- The clinical use of human culture-expanded autologous bone marrow mesenchymal stem cells transplanted on platelet-rich fibrin glue in the treatment of articular cartilage defects: A pilot study and preliminary results. Cartilage. 2010;1:253-61.
- [CrossRef] [PubMed] [Google Scholar]
- Effects of autogenous bone marrow aspirate concentrate on radiographic integration of femoral condylar osteochondral allografts. Am J Sports Med. 2017;45:2797-803.
- [CrossRef] [PubMed] [Google Scholar]
- The utility of biologics, osteotomy, and cartilage restoration in the knee. J Am Acad Orthop Surg. 2018;26:e11-25.
- [CrossRef] [PubMed] [Google Scholar]
- Analysis of outcomes for high tibial osteotomies performed with cartilage restoration techniques. Arthroscopy. 2017;33:486-92.
- [CrossRef] [PubMed] [Google Scholar]
- The quadriceps active ratio: A dynamic MRI-based assessment of patellar height. Eur J Orthop Surg Traumatol. 2018;28:1165-74.
- [CrossRef] [PubMed] [Google Scholar]
- Clinical accuracy of J-sign measurement compared to magnetic resonance imaging. Iowa Orthop J. 2016;36:94-7.
- [Google Scholar]
- The anatomy of the medial patellofemoral complex. Sports Med Arthrosc Rev. 2017;25:e8-11.
- [CrossRef] [PubMed] [Google Scholar]
- Variation in the medial patellofemoral ligament origin in the skeletally immature knee: An anatomic study. Am J Sports Med. 2017;46:1-7.
- [CrossRef] [PubMed] [Google Scholar]
- Medial quadriceps tendon-femoral ligament: Surgical anatomy and reconstruction technique to prevent patella instability. Arthrosc Tech. 2013;2:e125-8.
- [CrossRef] [PubMed] [Google Scholar]
- The effect of femoral tunnel position and graft tension on patellar contact mechanics and kinematics after medial patellofemoral ligament reconstruction. Am J Sports Med. 2014;42:364-72.
- [CrossRef] [PubMed] [Google Scholar]
- Allowing one quadrant of patellar lateral translation during medial patellofemoral ligament reconstruction successfully limits maltracking without overconstraining the patella. Knee Surg Sports Traumatol Arthrosc. 2018;26:2883-90.
- [CrossRef] [PubMed] [Google Scholar]
- MPFL reconstruction using a quadriceps tendon graft: Part 2: Operative technique and short term clinical results. Knee. 2014;21:1175-9.
- [CrossRef] [PubMed] [Google Scholar]
- Double-bundle anatomical versus single-bundle isometric medial patellofemoral ligament reconstruction for patellar dislocation. Int Orthop. 2013;37:617-24.
- [CrossRef] [PubMed] [Google Scholar]
- Influence of graft source and configuration on revision rate and patient-reported outcomes after MPFL reconstruction: A systematic review and meta-analysis. Knee Surg Sports Traumatol Arthrosc. 2017;25:2511-9.
- [CrossRef] [PubMed] [Google Scholar]
- The role of medial patellofemoral ligament repair and imbrication. Am J Orthop (Belle Mead NJ). 2017;46:87-91.
- [Google Scholar]
- Cartilage restoration techniques for the patellofemoral joint. J Am Acad Orthop Surg. 2017;25:321-9.
- [CrossRef] [PubMed] [Google Scholar]
- Cartilage restoration of patellofemoral lesions: A systematic review. Cartilage. 2019;2019:1947603519893076.
- [CrossRef] [PubMed] [Google Scholar]
- An algorithmic approach to the management of recurrent lateral patellar dislocation. J Bone Joint Surg Am. 2016;98:417-27.
- [CrossRef] [PubMed] [Google Scholar]
- Current use of navigation system in ACL surgery: A historical review. Knee Surg Sports Traumatol Arthrosc. 2016;24:3396-409.
- [CrossRef] [PubMed] [Google Scholar]
- Robotic and image-guided knee arthroscopy. In: Handbook of Robotic and Image-Guided Surgery. San Diego: Elsevier; 2019.
- [CrossRef] [Google Scholar]