Translate this page into:
The Posterior Cruciate Ligament: Anatomy, Biomechanics, and Double-Bundle Reconstruction
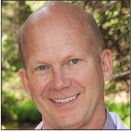
*Corresponding author: Robert F. LaPrade, M.D., Ph.D. Twin Cities Orthopedics, 4010 W 65th Street, Edina, Minnesota 55423 USA laprademdphd@gmail.com.
-
Received: ,
Accepted: ,
How to cite this article: LaPrade RF, Floyd ER, Falaas KL, Ebert NJ, Struyk GD, Carlson GB, et al. The posterior cruciate ligament: Anatomy, biomechanics, and double-bundle reconstruction. J Arthrosc Surg Sports Med 2021;2(2):94-107.
Abstract
The posterior cruciate ligament (PCL) is the largest intra-articular ligament in the knee and is the primary stabilizer to posterior tibial translation. Historically, the PCL’s functional dynamics and appropriate management after injury have been controversial. However, recent biomechanical and anatomic studies have elucidated a better understanding of PCL function, which has led to development of more anatomic reconstruction techniques. The larger anterolateral bundle and the smaller posteromedial bundle of the PCL exhibit a codominant relationship and have a wide femoral attachment footprint. For these reasons, the native kinematics of the knee is better restored with a double-bundle PCL reconstruction (DB-PCLR) technique than with a single-bundle PCL reconstruction (SB-PCLR). Likewise, clinical studies have demonstrated excellent outcomes for DB-PCLR compared to SB-PCLR, with decreased posterior knee laxity on stress radiography and improved International Knee Documentation Committee scores. This review will provide a detailed overview of the clinically relevant anatomy, biomechanics, injury evaluation, and treatment options, with an emphasis on arthroscopic DB-PCLR.
Keywords
Double-bundle
Knee
Posterior cruciate ligament
Posterior tibial translation
INTRODUCTION
Injury to the posterior cruciate ligament (PCL) has been reported to result in knee instability over time.[1] While these tears have been recognized as a source of pain and impaired function, they have also been reported to lead to the development of osteoarthritis.[1]
However, the actual prevalence of PCL injury is relatively unknown given that this injury is often misdiagnosed or undiagnosed.[2,3] Current PCL injury prevalence data vary greatly (1– 44%) based on different study settings and populations. [4-9] Thus, a proper understanding of PCL injury is of clinical importance in the practice of orthopedic surgery and sports medicine.
The understanding of PCL anatomy and biomechanics has greatly improved in recent years and has resulted in improved conservative, surgical, and rehabilitation treatment options.[10-14] However, controversy remains over decision-making for non-operative versus operative care, and the choice of the appropriate surgical technique to provide the best clinical outcomes.[10,12,15-20] Current literature is limited regarding comparative PCL surgical techniques and long-term follow-up studies.[10] This review will discuss the current state of knowledge of PCL anatomy and biomechanics, diagnosis and treatment modalities, and the authors’ preferred surgical technique and associated clinical outcomes.
ETIOLOGY/INCIDENCE
Isolated PCL tears are relevantly rare, comprising only 3% of outpatient knee injuries.[5] These injuries more commonly occur alongside other ligament tears (approximately 97%), and constitute up to 38% of acute knee injuries at a trauma center.[5] Injury to the PCL classically occurs due to a “dashboard injury” or external trauma from a posterior force applied to the anterior aspect of the proximal tibia during knee flexion. PCL tears also commonly occur with a fall onto the knee with a plantar-flexed foot or with a direct, posteriorly directed strike to the anterior tibia, as is commonly seen in athletic injuries during American football, skiing, rugby, and soccer/football.[5] Non-contact PCL injury due to hyperextension or hyperflexion is much less common.[21]
ANATOMY
The PCL is the largest intra-articular ligament in the knee. It originates on the anterolateral aspect of the medial femoral condyle and inserts inferior to the posterior joint line into a depression between the posterior aspects of the medial and lateral tibial plateaus, called the PCL facet, and just proximal to a shallow ledge known as the champagne-glass drop-off.[22-25] The mean length of the PCL ranges from 32 mm to 38 mm, while the mean cross-sectional area ranges from 11 mm2 to 13 mm2.[24,26] It is composed of two distinct but inseparable bundles, the larger anterolateral bundle (ALB) and the smaller posteromedial bundles (PMB).[24,27-29]
Femoral attachment of the PCL
The area of the femoral attachment of the PCL in the medial intercondylar notch is approximately double the size of its tibial attachment and has been reported to range from 112 mm2 to 118 mm2.[22,28,30,31] The anatomic centers of the ALB and PMB femoral attachments are an average of 12.1 mm apart. Several arthroscopic landmarks have been described to aid the surgeon in determining the femoral attachments of the PCL bundles; these are the trochlear, medial arch, and posterior points along the condylar cartilage margin, and the medial intercondylar ridge and bifurcate prominence of the intercondylar notch [Figure 1].

- Femoral anatomy of the posterior cruciate ligament (PCL). The anterolateral bundle (ALB) and posteromedial bundle (PMB) are visible, surrounded by bony reference points: the trochlear, medial arch, and posterior points. The anterior footprint of the ALB extends between the trochlear and medial arch points. The medial intercondylar ridge (dashed line) forms the proximal border of the ALB and PMB, and extends anteriorly across the medial intercondylar notch from the posterior point. The tibial attachment of the PCL has been sectioned and the tibia removed in (a). (b) demonstrates the native orientation of the PCL fibers in flexion.
The ALB is located along the roof of the intercondylar notch and adjacent to the articular cartilage margin. The center of the ALB is located 7.4 mm from the trochlear point, 11.0 mm from medial arch point, and 7.9 mm from the nearest point on the distal articular cartilage.[14,22,32] The medial intercondylar ridge constitutes the proximal borders of the ALB and PMB femoral attachments.[22,28,33] Some authors have described a medial bifurcate ridge that separates the two bundles, but the incidence of this structure is variable.[22,28,32,34]
The smaller PMB femoral footprint is located along the wall of the notch between the footprints of the anterior meniscofemoral ligament (aMFL) and posterior meniscofemoral ligament (pMFL) attachments and has an area that ranges from 60 to 90 mm2.[22,28,32] Its center is located 11.1 mm from the medial arch point, 10.8 mm from the posterior point of the articular cartilage margin, and 8.6 mm posterior to the distal articular cartilage margin.[22] The PMB femoral footprint is bordered anteriorly by the ALB and proximally by the medial intercondylar ridge.[22,28,32] It was noted by Anderson et al. that the distal border of the PMB is usually shared with the aMFL, if it is present.[22]
Tibial attachment of the PCL
In comparison to its femoral attachment, the PCL tibial insertion is more compact.[23,35,36] The fibers of the two bundles insert together below the articular surface onto the PCL facet, roughly 50% across the width of the tibial plateau.[23,25] At the tibial attachment, the PMB footprint wraps posteriorly around the ALB footprint in an analogous pattern to how the AMB of the ACL wraps anteriorly around the PLB.[22,32,37] A bony landmark, termed the bundle ridge, lies posterior to the ALB insertion and anterior to the PMB, separating the tibial footprints of the two bundles.[38,39] During PCL reconstruction, the drill guide should be positioned so that the guide pin emerges just proximal (1.3 mm) to the bundle ridge[22] [Figure 2]. Due to the compact nature of the tibial PCL attachment, only one tibial tunnel is necessary.
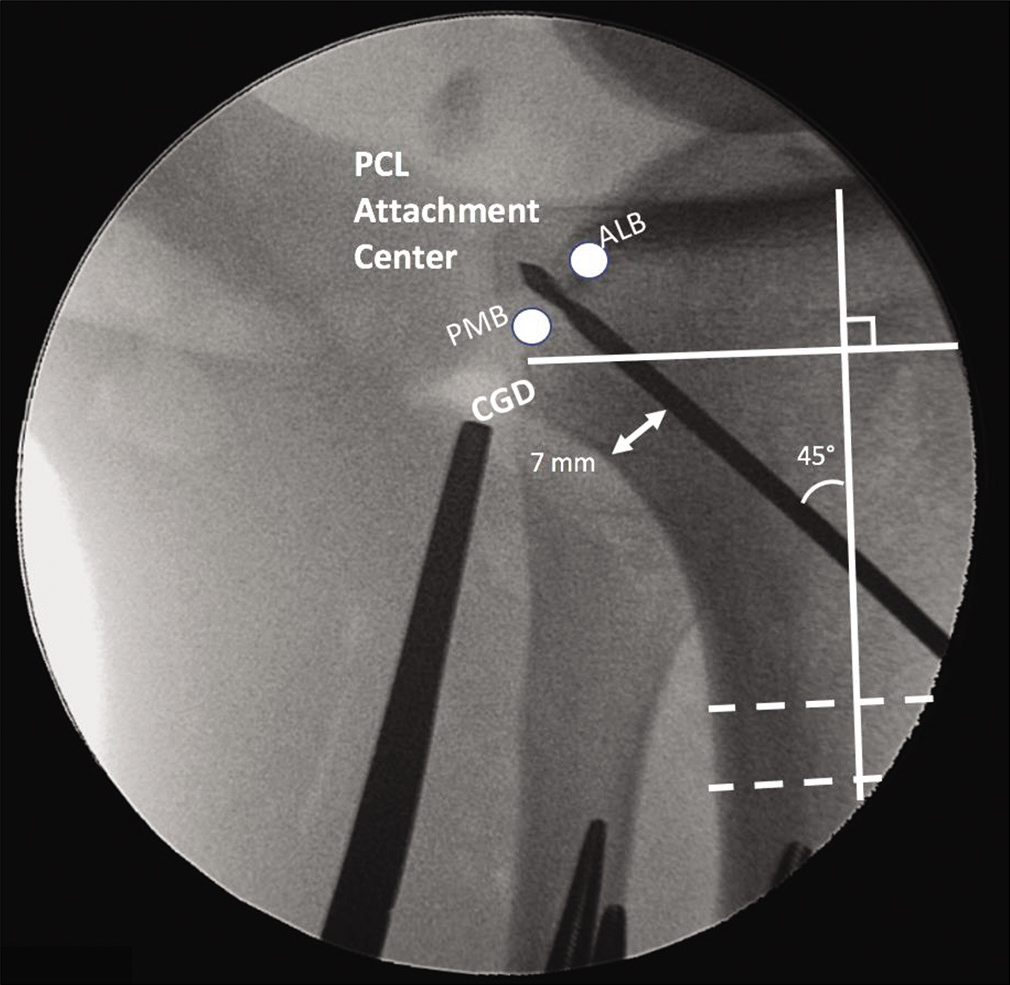
- Fluoroscopic evaluation of tibial guide pin placement during posterior cruciate ligament (PCL) reconstruction. The grafts for the anterolateral bundle and posteromedial bundle (ALB and PMB) grafts are secured in two femoral tunnels. An Achilles tendon allograft is used for the ALB and a tibialis anterior allograft for the PMB. Both grafts then pass through one transtibial tunnel. A guide pin is placed before creating the tibial tunnel, entering midway between the anterior tibial crest and the medial border of the tibia and emerging between the anatomic ALB and PMB centers on the tibial PCL facet, indicated above with white circles. The PCL facet is just superior to the champagne-glass drop-off (CGD), and the footprints of the bundles are separated by a bundle ridge. A 7 mm back wall should be maintained. The tibial tunnel is drilled at a 45° angle with the long axis of the tibia, perpendicular to two horizontal lines (dashed lines) from the anterior to posterior tibial cortices. a
The distolateral corner of the shiny white fibers (SWFs) of the posterior horn of the medial meniscus (PHMM) has been termed the SWF point and is one of several PCL facet arthroscopic landmarks. It is important to not impinge on the SWFs during PCL tunnel reaming to avoid an iatrogenic medial meniscus root tear [Figure 3].
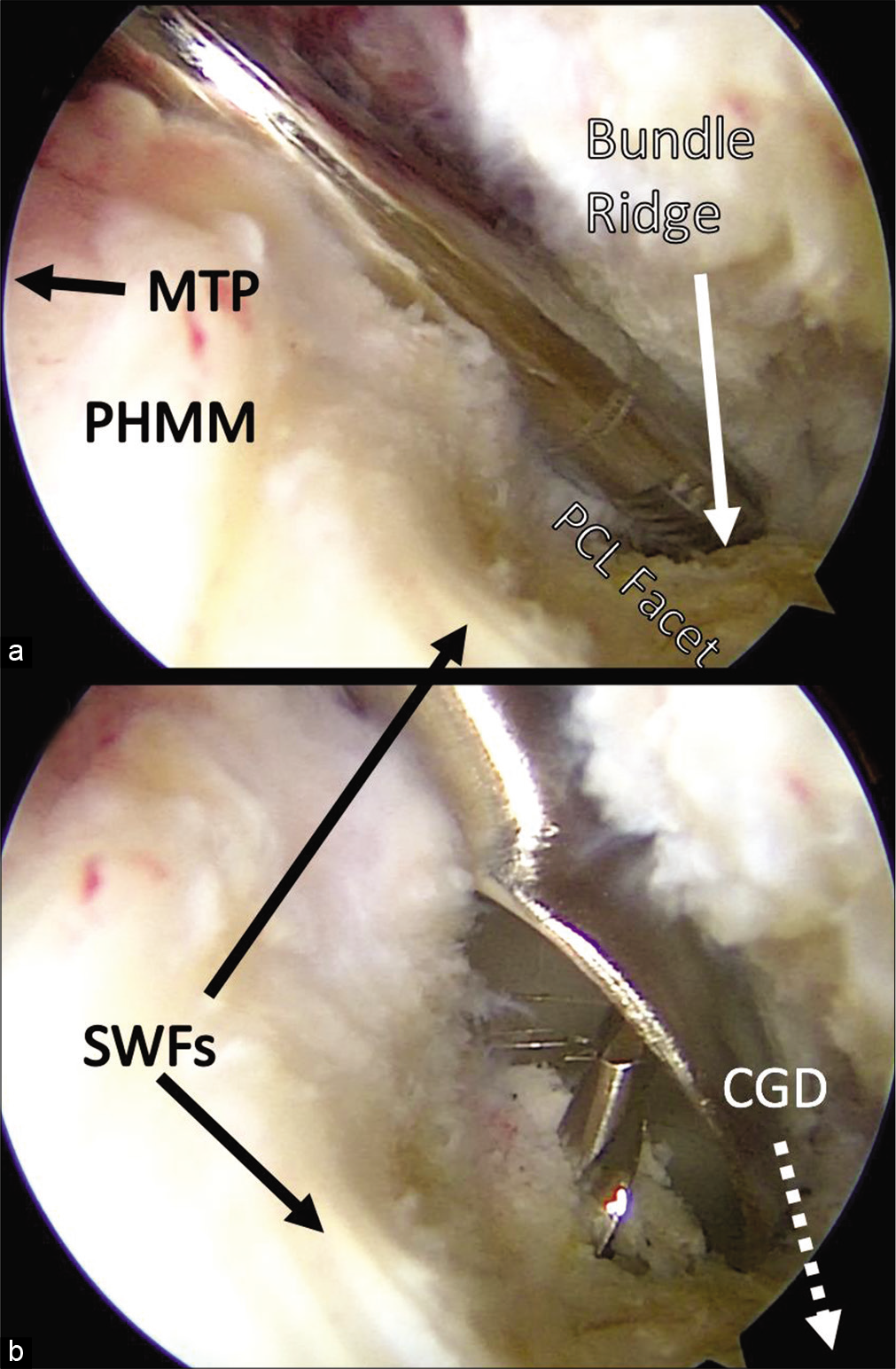
- Posterior cruciate ligament (PCL) facet arthroscopic anatomy: These two views from the posteromedial portal demonstrate important landmarks during PCL reconstruction. In (a), an arthroscopic shaver is used to clean the area around the tibial PCL insertion after ablation. The tip of the shaver can be seen dipping behind the bundle ridge, a structure which separates the insertions of the anterolateral bundle and posteromedial bundle. In (b), the shiny white fibers (SWFs) of the medial meniscus can be seen as the tibial guide pin emerges from the PCL facet and a large curette protects from over-penetration posteriorly. PHMM: Posterior horn of the medial meniscus, MTP: Medial tibial plateau, CGD: Champagne-glass drop-off.
Radiographic description of PCL tibial anatomy can be divided into anteroposterior (AP) and lateral views. On the AP view, the PCL anatomic center is located 1.6 mm ± 2.5 mm distal to the joint line. For medial-lateral reference, the PCL tibial insertion is distally aligned with the lateral tibial eminence (LTE).
On the lateral radiographic view, the center of the PCL footprint lays 5.5 ± 1.7 mm superior to the perpendicular champagne glass drop-off (CGD) line. The CGD line refers to a reference line drawn perpendicular to the long axis of the tibia which intersects the CGD on the posterior tibial cortex[38,39] [Figure 4].

- Lateral and anteroposterior (AP) radiography of the posterior cruciate ligament (PCL): (a) Lateral tibia view. The measurement axis is determined by drawing the longitudinal axis of the tibia and calculating distances from a perpendicular line intersecting with the champagne-glass drop-off (CGD). (b) The AP measurements are taken from a line traversing the proximal joint line, and a perpendicular line intersecting the apex of the medial tibial eminence (MTE). ALB: Anterolateral bundle, PMB: Posteromedial bundle, LTE: Lateral tibial eminence.
Meniscofemoral ligaments
The meniscofemoral ligaments are closely associated with the PCL bundles and connect the posterior horn of the lateral meniscus to the intercondylar notch[23,26,40] [Figure 5]. The anterior meniscofemoral ligament (of Humphrey) and the posterior meniscofemoral ligament (of Wrisberg) cross anteriorly and posteriorly to the PCL, respectively.[23,26,40,41] The aMFL has a been reported to be present in 74–75% of knees and the pMFL has been reported in 59–80% of knees. [22,29,41,42] The pMFL femoral attachment is found proximal to the PMB and the medial intercondylar ridge.[22] Ideally, the MFLs should be left intact during a DB-PCLR [Figure 6].
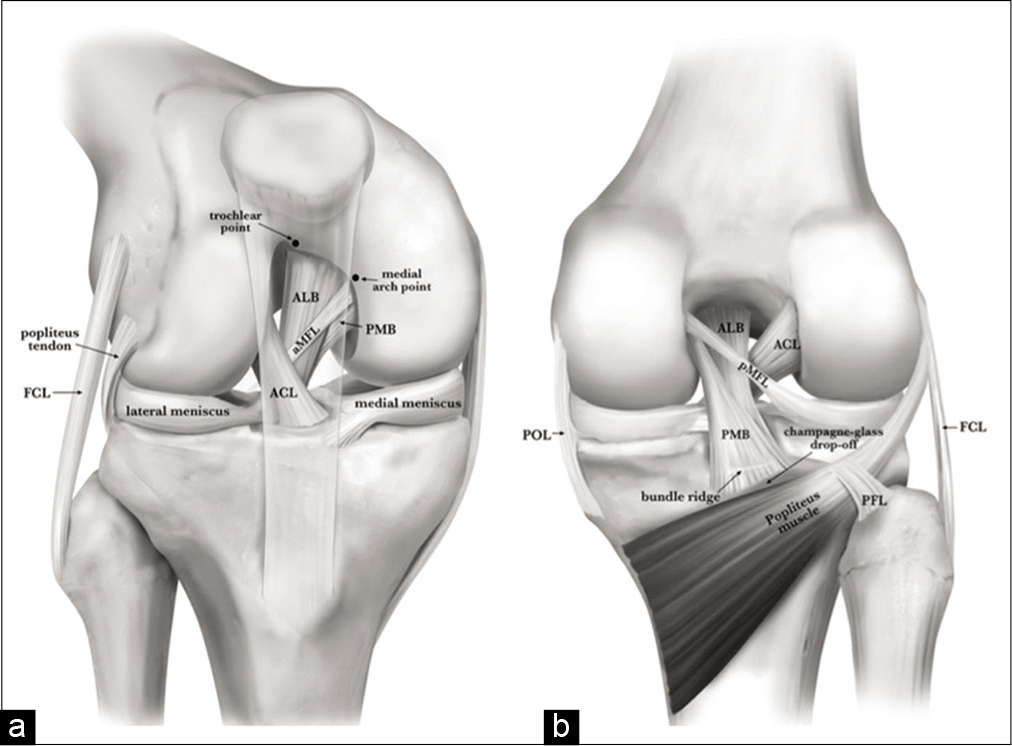
- Anterior (a) and posterior (b) anatomy of the posterior cruciate ligament (PCL). The anterior border of the anterolateral bundle (ALB) is visible between the trochlear and medial arch points (a). The posteromedial bundle (PMB) fibers wrap around the tibial insertion of the ALB fibers in (b). The anterior meniscofemoral ligament (aMFL) attaches to the intercondylar notch anterior to the PMB, while the posterior meniscofemoral ligament (pMFL) inserts posterior to the PMB. In (b), the tibial landmarks of the PCL facet are also visible. The bundle ridge separates the tibial footprints of the ALB and PMB, just above the champagne-glass drop-off (CGD). Reproduced with permission from Kennedy NI, Wijdicks CA, Goldsmith MT, et al. Kinematic analysis of the posterior cruciate ligament, Part 1: The individual and collective function of the anterolateral and posteromedial bundles. Am J Sports Med. 2013;41(12):2828-2838.
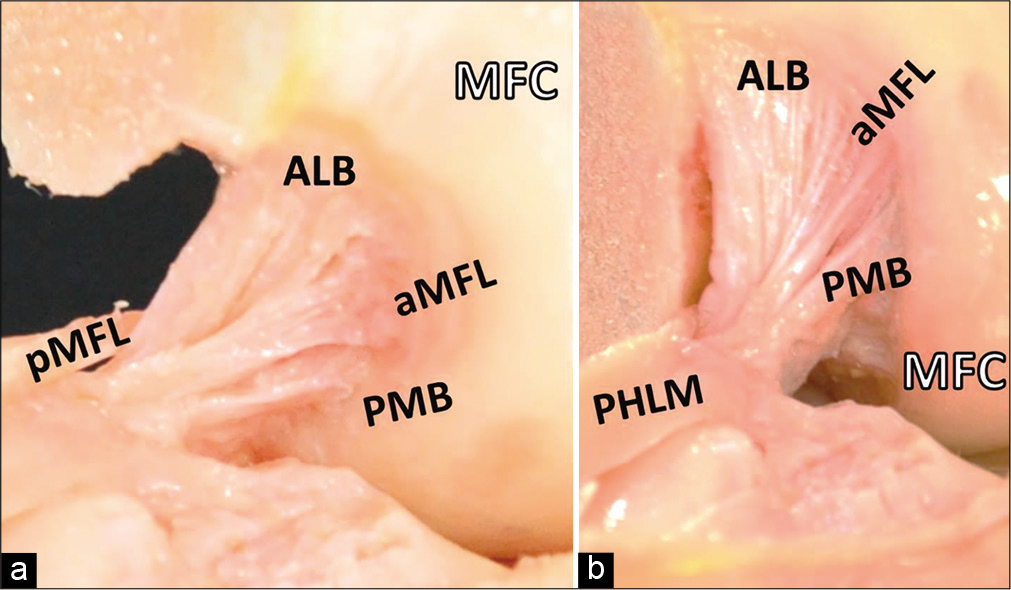
- Intercondylar notch attachments of the posterior cruciate ligament (PCL), and anterior meniscofemoral ligament and posterior meniscofemoral ligament (aMFL and pMFL) attachments. The pMFL passes posteriorly to the PCL from the posterior horn of the lateral meniscus (PHLM), while the aMFL passes anteriorly. The femur has been sectioned in this view, leaving only the medial femoral condyle (MFC) to demonstrate the medial intercondylar notch. (a) The knee in 90° and (b) 130° of flexion.
BIOMECHANICS
The main biomechanical functions of the PCL are to provide restraint against posterior tibial translation (PTT) at all flexion angles, and limit internal and external rotation beyond 90° of flexion.[13,31] The PCL, in addition to being the largest intra-articular ligament, is also the strongest due to its high tensile strength (1620 N and 258 N for ALB and PMB, respectively) and fiber orientation.[13,43,44] The anterolateral and PMB were originally thought to exhibit reciprocal independent function, with the ALB predominant in deep flexion, and the PMB predominant in extension.[22,27,45,46] However, recent literature has shown that the PCL bundles together exhibit synergistic and codominant behavior throughout the range of motion (ROM) of the knee.[31] Both the ALB and PMB provide resistance to PTT throughout all flexion angles and the PCL as a whole has been reported to contribute up to 95% of posterior knee stability between 30° and 90° of knee flexion.[27,30,47,48]
A recent study observed 11.7 mm of PTT at 90° after complete PCL sectioning (simulating a Grade III PCL tear).[31] Additional biomechanical studies have also reported that the PCL plays a more varied role in rotational stability than has historically been appreciated. Kennedy et al., reporting on 20 match-paired human cadaveric knees, found that the PCL restricted internal rotation at all ranges of flexion, with the PMB specifically providing the majority of rotational control beyond 90° of flexion.[31]
Fiber orientation and length within each respective PCL bundle corresponds to specific ranges of knee flexion; the ALB fibers are horizontal in full extension and become more vertically oriented in higher degrees of flexion. By contrast, the fibers of the PMB are more vertical in extension and horizontal in flexion [Figure 7].[13,30,45] The ALB acts as the main restraint to PTT at higher degrees of knee flexion (70° and 105°), and the PMB acts as the main restraint to PTT close to extension (0° and 15°).[13] As has been noted, this interplay of fiber orientation and length ensure that neither bundle predominates and both are integral to preventing PTT throughout the ROM.[13]
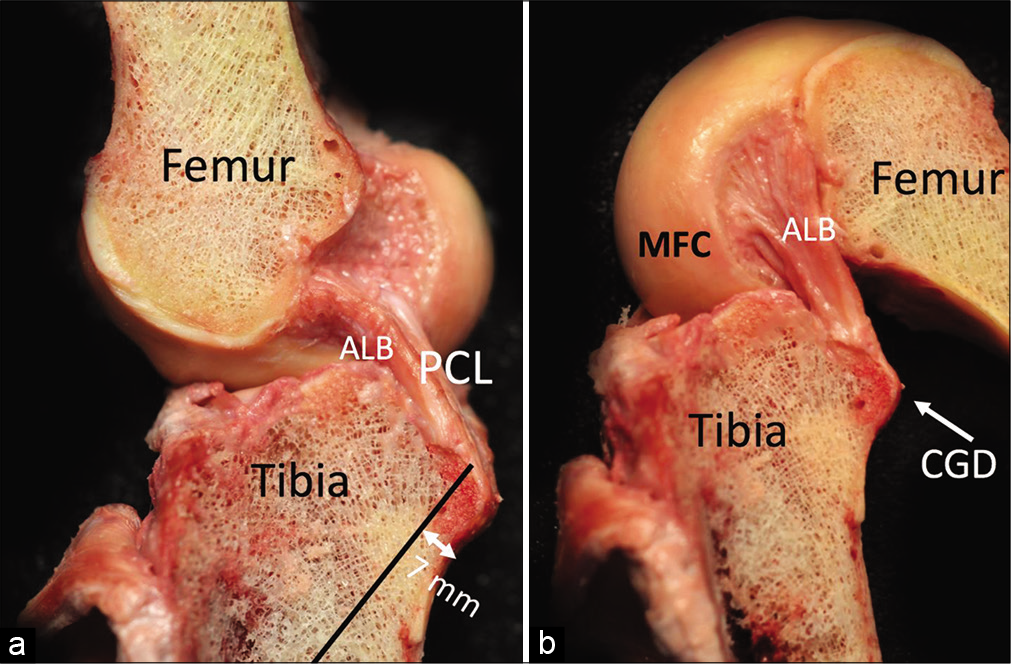
- Orientation of the posterior cruciate ligament (PCL) fibers in extension and in flexion. The fibers of the anterolateral bundle (ALB) are more horizontal in extension (a) and vertical in flexion (b). By contrast, the posteromedial bundle (PMB) fibers are more vertical in extension and horizontal in flexion. MFC: Medial femoral condyle.
OPERATIVE MANAGEMENT
Diagnosis and evaluation
A majority of PCL tears occur concurrently with other injuries, most commonly to the posterolateral corner (PLC).[32,49-54] The patient history should clarify the time of onset, mechanism of injury, and associated symptoms.
Patients with isolated PCL injuries often may be uncertain of the initial injury, while patients with combined or multi-ligament injuries tend to remember an inciting event.[55] The physical exam should include inspection for a posterior sag sign. Maneuvers should include posterior drawer testing, varus and valgus stress, posterolateral and posteromedial drawer tests, quadriceps active test, and the external rotation recurvatum test.[32,50,51,55] Finally, AP radiographs, stress radiographs, and magnetic resonance imaging (MRI) are used to confirm the diagnosis and to determine the presence of an isolated versus combined PCL injury[56] [Figures 8-10]. MRI is generally considered the gold standard in diagnosis of acute PCL tears, because it has a sensitivity of 100% and specificity of 97–100% in the acute phase.[32,55,57,58] However, recent studies have reported that MRI is less sensitive and specific when assessing for a chronic PCL tear.[32,50,55,59,60] This may be due to an accumulation of scar tissue, or healing in a non-functional and elongated position, which may hide the extent of damage to the ligament.[57,61]
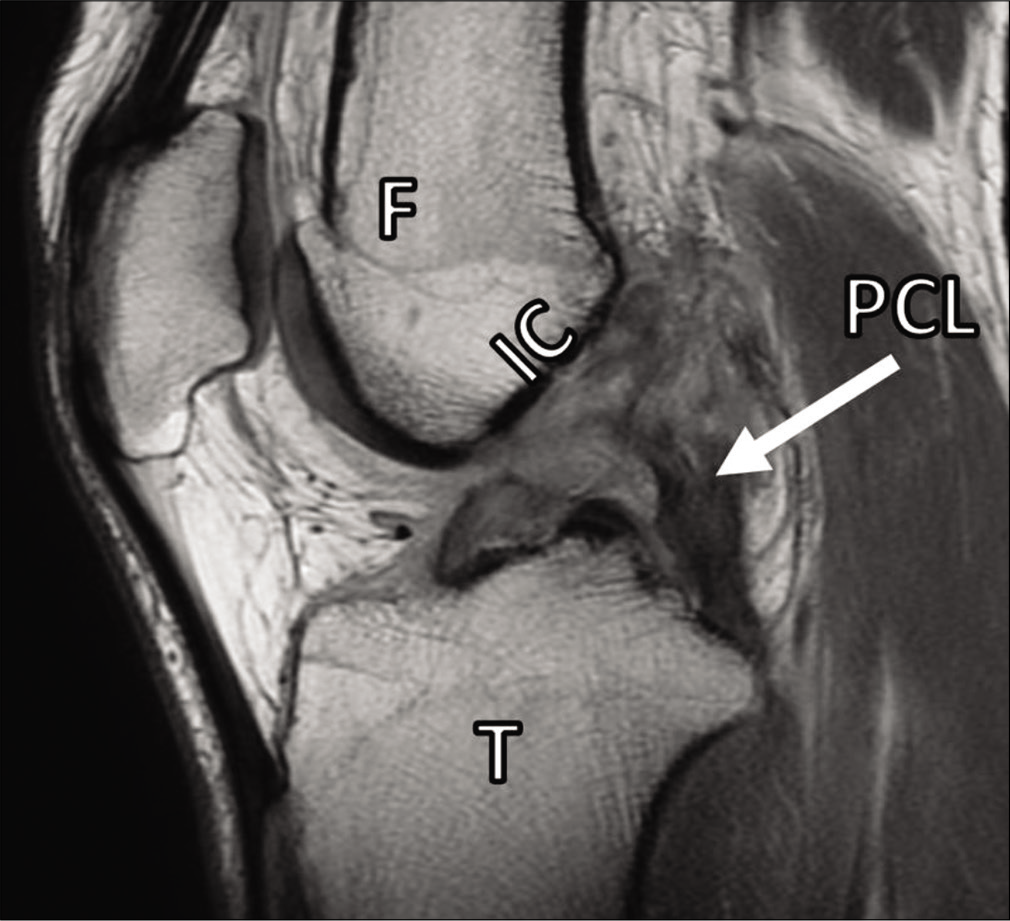
- Sagittal proton dense magnetic resonance image demonstrating a complete rupture of the posterior cruciate ligament (PCL, white arrow). Grade III injury of the PCL occurs when fibers of the PCL are completely disrupted. The posterior drawer test may be used to detect increased posterior translation on physical examination. The patient in this image also has an anterior cruciate ligament injury. F: Femur, T: Tibia, IC: Roof of intercondylar notch.
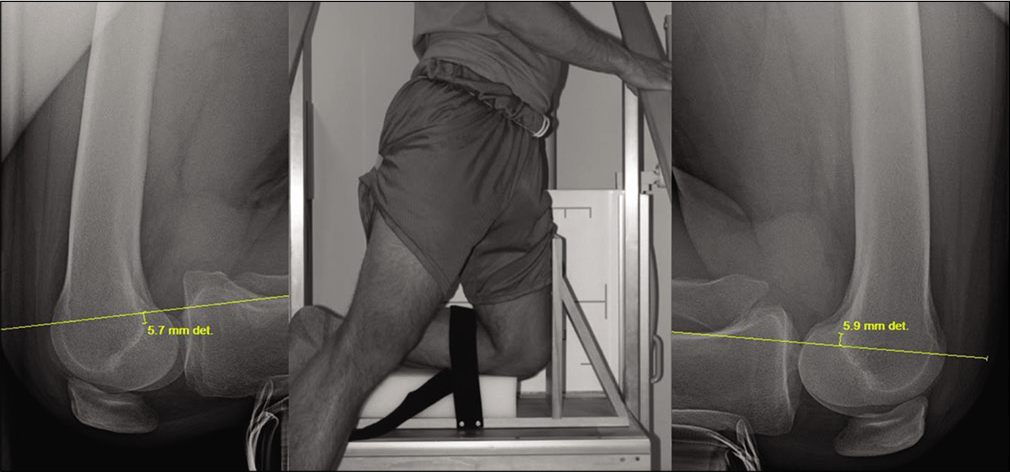
- Lateral kneeling posterior stress radiographs are used to measure side to side differences in translation of the femur over the tibia to assess the integrity of the posterior cruciate ligament. In the center insert, an example apparatus is shown for taking posterior stress radiographs; the proximal edge of the patient’s tibial plateau rests on the box. To measure posterior stress radiographs, one line is drawn along the posterior cortex of the tibia, starting from at least 15 mm distal to the joint line. The perpendicular distance is measured from this line to the posterior-most point of Blumensaat’s line for each knee. The sum of these differences from both knees is the amount posterior tibial translation (PTT). In this example, that distance would be 11.6 mm of PTT (5.7 mm from one knee added to 5.9 mm from the other knee).
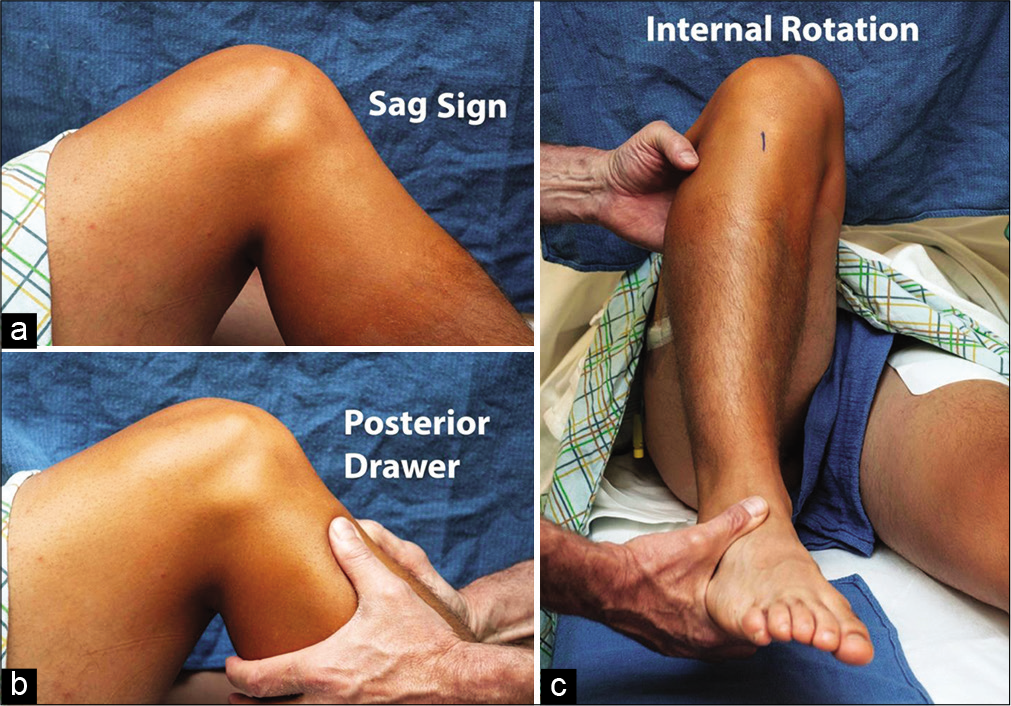
- Some physical exam maneuvers to evaluate posterior cruciate ligament (PCL) injuries. (a) The sag sign is observed as the knee is flexed to 90° with the hip flexed to 45° and the tibia appears to hang loosely posteriorly. (b) The posterior drawer test is performed with the hip and knee as above, and direct posteriorly-directed pressure is applied. In a normal knee, there should be no posterior translation. The knee should be totally relaxed and can be stabilized in neutral rotation by the examiner sitting on the foot. Grades I, II, and III tears can be roughly estimated by judging whether the tibia remains anterior to the femoral condyles, slips to the level of the condyles, or falls posterior to the condyles, respectively. (c) An increased side to side difference in the supine internal rotation test is also highly sensitive and specific for a Grade III PCL tear. Reprinted with permission from: Moulton SG, Cram TR, James EW, Dornan GJ, Kennedy NI, LaPrade RF. The supine internal rotation test: A pilot study evaluating tibial internal rotation in Grade III posterior cruciate ligament tears. Orthop J Sports Med 2015;3(2):2325967115572135. Published 2015 Feb 23.
Kneeling posterior stress radiographs measure side-to-side differences in PTT and are an objective, validated tool for determining posterior laxity, isolated versus combined injury, and for assessing chronic injuries.[32,49,50,62,63] Partial PCL tears result in <8 mm of PTT (Grade 1), isolated complete PCL tears result in 8–12 mm of increased PTT (Grade 2), and PTT of >12 mm indicates a complete tear with injuries to other ligaments (Grade 3).[32,50] The degree of tibial translation is important in determining the appropriate treatment for PCL tears. Patients with <8 mm of posterior translation may consider a non-operative rehabilitation program, while patients with more than 8 mm of PTT are more likely to require reconstructive surgery.[32,64] We recommend that all surgeons performing PCLR obtain both pre-operative and post-operative PCL stress radiographs to objectively assess the degree of injury and their post-operative outcomes [Figures 9 and 10].
NON-OPERATIVE MANAGEMENT
The PCL has some intrinsic healing ability due to its blood supply from the adjacent middle geniculate artery, which passes the PCL insertion on its way to the joint capsule.[32,65,66] Therefore, some Grades 1 and 2 isolated PCL injuries may be treated using a non-operative rehabilitation program. Isolated PCL tears may be characterized by <8 mm difference in posterior laxity compared to the contralateral knee, <5° abnormal internal or external rotation at 30° of flexion, and an absence of concomitant collateral ligament injury.[67] A non-operative management program should emphasize strengthening of the quadriceps mechanism and include the use of a dynamic PCL brace that returns the knee into neutral position and prevents posterior sag of the tibia.[32] Often, some residual laxity has been reported after non-operative treatment due to healing in an attenuated position.[32,60] Outcome studies after non-operative management of isolated PCL tears have reported that objectively measured degrees of laxity often do not correlate with severity of symptoms.[8,68,69] A prospective study by Shelbourne et al. (1999) examining a cohort of 133 nonoperatively treated patients with isolated PCL injuries reported improved Lysholm, Tegner, and modified Noyes subjective stability scores for pain and activity. Shelbourne et al. correlated improved Noyes subjective stability scores with improved quadriceps strength, but only roughly half of patients treated according to their non-operative regimen returned to sports at or near previous levels.[8] However, Shelbourne’s study did not obtain initial or final PCL stress radiographs so objective data are lacking. Other authors have reported that patients treated nonoperatively have higher residual laxity by objective measurement with MRI and stress radiographs compared to those treated by surgical reconstruction.[17,32,64] Patients with PTT >8 mm or those with concomitant multi-ligament injuries may be better managed surgically to restore knee function and decrease the risk of future knee osteoarthritis.[17,32,50]
SINGLE-BUNDLE PCL RECONSTRUCTIONS (SB-PCLR) VERSUS DOUBLE-BUNDLE PCL RECONSTRUCTIONS (DB-PCLR)
Historically, SB-PCLR and DB-PCLR have been reported and practiced without a clear understanding of the biomechanics and functional anatomy of the PCL. In a SB-PCLR technique, only the ALB is reconstructed, while DB-PCLR restores the ALB and PMB with two femoral reconstruction tunnels. While both procedures have been reported to be successful with functional scores at short-term follow-up, biomechanical studies have clearly found that DB-PCLR provides practically native knee kinematic restoration, greater rotational stability, and normal anatomy in addition to higher International Knee Documentation Committee (IKDC) scores and decreased PTT.[22,30-32,70-74] Therefore, the authors’ preferred surgical technique is a DB-PCLR with an 11 mm Achilles tendon allograft for the ALB reconstruction, and a 7 mm tibialis anterior allograft for the PMB reconstruction. In locations or for patients where allografts are not desired or available, we have found that the ALB can be reconstructed with a quadriceps tendon autograft with a bone plug and a semitendinosus autograft for the PMB.
Two-stage procedures have been reported using proximal tibial osteotomies in some patients with proximal tibial slope or varus/valgus deformities. A shallow proximal tibial slope (PTS, <5–6°) may cause increased AP instability and laxity, and corrective slope increasing proximal tibial osteotomy may be considered as a first stage for chronic PCL injuries to decrease shear forces on the graft.[75] In patients with combined chronic PCL and PLC injuries, a biplanar osteotomy needs to be considered to correct additional varus malalignment before multiple ligament reconstructions due to the high risk of failure of the PLC reconstruction grafts. [50,75,76]
SURGICAL TECHNIQUE
The patient is first placed supine on the operative table and induced with general anesthesia. A bilateral knee examination should be performed to confirm the diagnosis, assess the ROM, and evaluate for any concurrent ligament insufficiency. The tibiofemoral step-off should be assessed in the normal contralateral knee as the native tibiofemoral relationship should be restored by the reconstruction. The operative leg should then have a well-padded high thigh tourniquet placed, while the contralateral knee is placed in an abduction stirrup.
An Achilles tendon allograft with a 20 mm long and 11 mm in diameter calcaneal bone plug is used for the ALB graft.[50] The opposite end of the graft is tubularized with a No. 5 nonabsorbable suture to pass through the tibial bone tunnel. A tibialis anterior tendon allograft is used for the PMB, and tubularized at both ends with non-absorbable sutures, the proximal end sized to fit into a 7 mm femoral tunnel.
Standard arthroscopy is performed with anterolateral and anteromedial entry portals, using the bony landmarks in the intercondylar notch. The anterior aspect of the ALB femoral attachment anterior is located near the trochlear point, while the posterior ALB attachment site is found at the medial arch point.[22] The femoral footprint of the PMB is found distal to the medial arch point and along the wall of the notch, approximately 8–9 mm posterior to the medial femoral condyle’s articular cartilage edge[22] [Figure 1]. These sites are then marked with an arthroscopic coagulator (Smith and Nephew, Andover, MA).
Next, the guide pins are drilled at the anatomic centers of the ALB and PMB bundles. The ALB tunnel is kept as distal as possible, against the edge of the articular cartilage. Each pin is then overreamed to a depth of 25 mm over its guide pin, the ALB with an 11 mm reamer and the PMB with a 7 mm reamer with a 2 mm bone bridge between the tunnels, the PMB tunnel is notched at the posteroinferior aspect to prevent later difficulty with interference screw placement, and passing sutures are placed [Figure 11].

- Femoral tunnels for double-bundle posterior cruciate ligament reconstruction. Guide pins (a) are first drilled through the anatomic centers of the anterolateral bundle (ALB) and posteromedial bundle (PMB). The guide pins are overreamed with an 11 mm reader to create an ALB tunnel, and a 7 mm reamer to create a PMB tunnel (b). The eyelet pins are then used to place passing stitches for later use in graft passage. It is important to leave at least 2 mm between the tunnels to prevent tunnel breakthrough. MFC: Medial femoral condyle.
Attention is then turned toward identification of the PCL tibial attachment. Using arthroscopic shavers and coagulators, the PCL tibial footprint is carefully exposed through a posteromedial arthroscopic portal, with consideration for the proximity of nearby popliteal neurovascular structures. The SWFs of the PHMM are identified, and the dissection continued posterolaterally to identify the bundle ridge. The tibial attachment site of the PCL is located distal to the joint line, in the PCL facet just above the CGD. A guide pin is then drilled through the anteromedial tibia, entering the anteromedial tibia between the medial tibial border and the anterior tibial crest, 6 cm distal to the joint line. It should exit posteriorly at the bundle ridge in the center of the PCL tibial attachment.[22] Intraoperative fluoroscopy is used to verify correct tibial pin placement. AP views should demonstrate this pin 1–2 mm distal to the joint line, in line with the medial aspect of the LTE.[50] On lateral views, the pin should emerge 6–7 mm proximal to the champagne-glass drop-off[50] [Figure 2].
The tibial tunnel is then reamed using a 12-mm acorn reamer to over-ream the guide pin. Reaming the tibial tunnel too proximally carries a risk of iatrogenic medial meniscal root injury. Complete meniscal root injury results in altered joint biomechanics identical to that seen with a medial meniscectomy and should be avoided at all costs.[77-80] It should also be noted that a smooth-bore reamer is ill-advised during tibial tunnel reaming due to a high risk of iatrogenic popliteal artery injury from unknown posterior tibial cortex penetration.[81] It is recommended to use a large curette to prevent migration of the guide pin during tibial reaming and that the surgeon lower their hand while approaching the tibia’s posterior cortex to allow the acorn reamer to “walk over” the posterior cortex, especially if bone chatter is encountered. The curette prevents over-penetration and protects the popliteal artery from injury. The reamer’s exit from the posterior tibial cortex is usually performed by hand. Use of a smoother device (Gore Smoother, Smith and Nephew, London, UK) will help clean the aperture and ease graft passage; the smoothing tool also functions as the tibial passing stitch.[50] If PCLR is performed in the setting of a multi-ligament reconstruction, it is important to avoid convergence of numerous reconstruction tunnels through the femur and tibia.[82-84]
Graft passage should then be performed. The PMB allograft should first be passed into the PMB femoral tunnel through the anterolateral arthroscopic portal with the help of the passing suture and is then fixed with a 7 × 20 mm bioabsorbable interference screw (Smith and Nephew, London, UK) positioned at the tunnel’s posteroinferior aspect [Figure 12]. Next, the ALB’s bone plug is similarly passed into its respective femoral tunnel. The cortical side of the bone plug should be set in the ALB femoral tunnel’s anterior portion and alongside the articular cartilage. The graft is then secured with a 7 × 20 mm titanium interference screw positioned at the tunnel’s anterosuperior aspect.
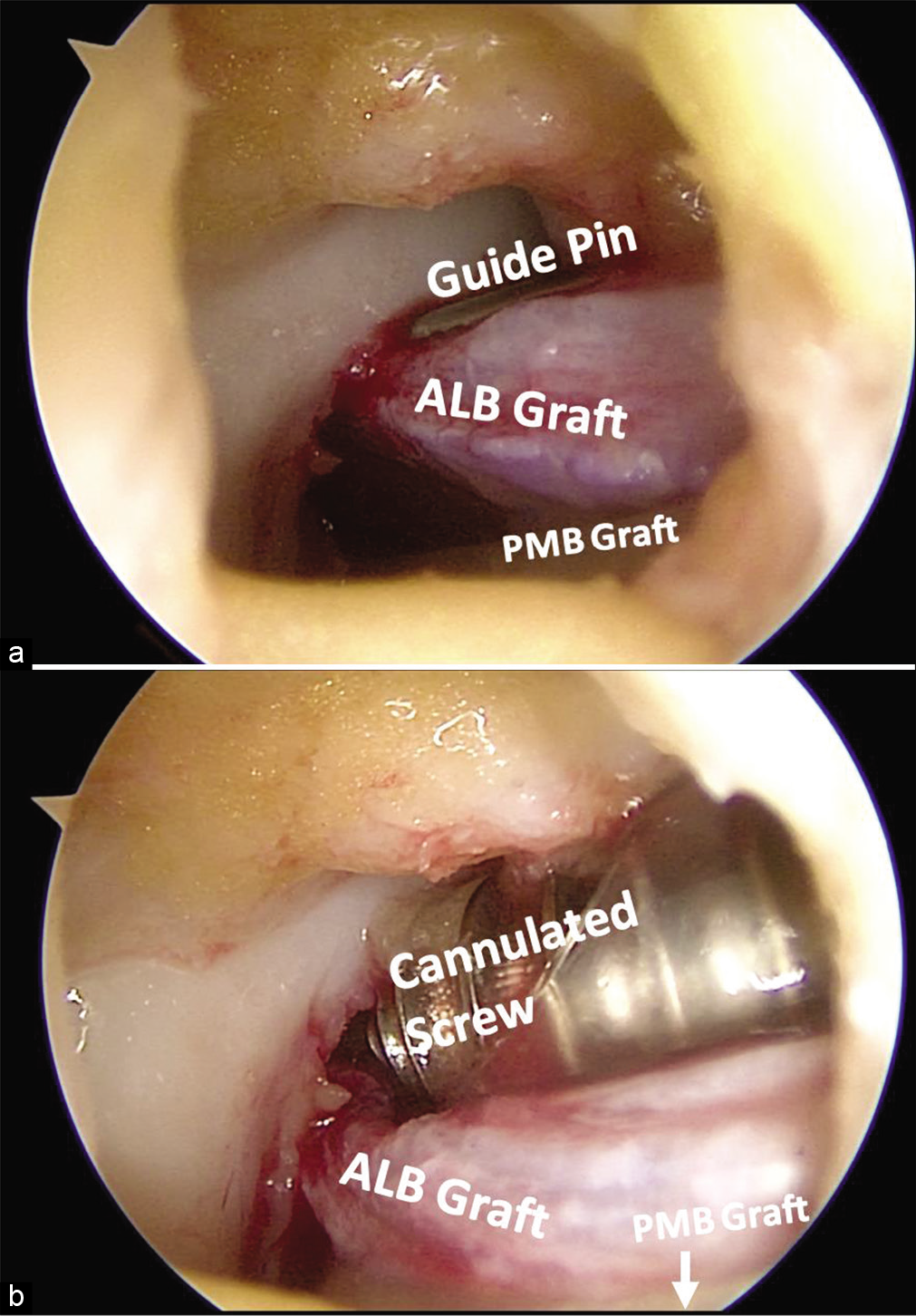
- Securing the PCL graft in the femur using interference screws. A 7 x 20 mm bioabsorbable screw is first used to secure the tibialis anterior graft in the posteromedial bundle (PMB) tunnel. Next, a 7 x 20 mm titanium cannulated screw is passed over a guide pin (a) to secure the Achilles tendon and with bone plug in the anterolateral bundle (ALB) tunnel. The titanium screw is passed through a plastic cannula to avoid soft tissue bridging during fixation (b) to secure the ALB bundle graft.
The sutures in the ends of both grafts are then passed distally into the tibial tunnel using the aforementioned smoothing tool and out of the anteromedial aspect of the tibia, after PCL graft femoral fixation [Figure 13]. The grafts should be individually cycled multiple times to avoid bunching up of the grafts, followed by arthroscopic verification of appropriate reduction. Normal tibiofemoral step-off should also be assessed.
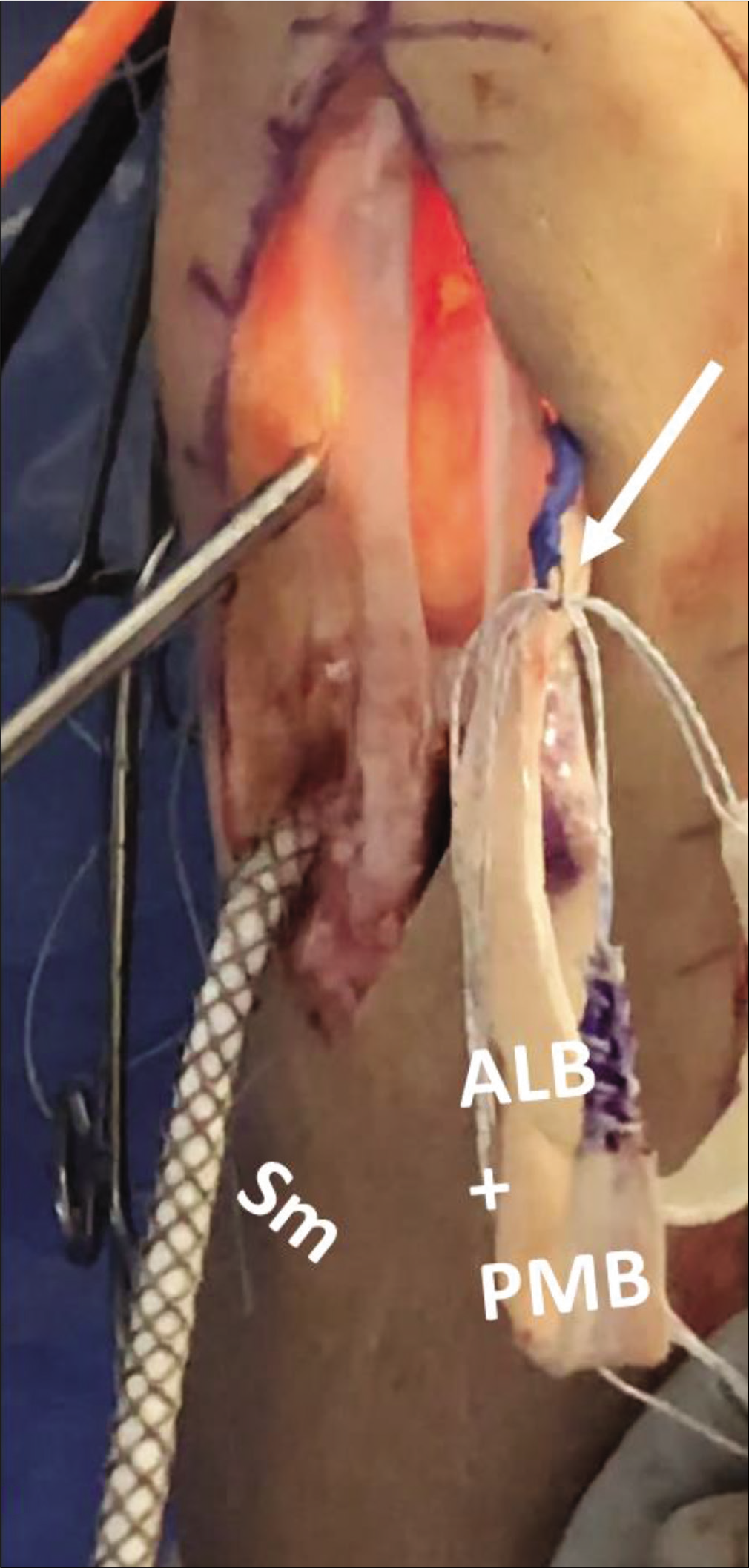
- Graft passage through the tibial tunnel. After securing the proximal anterolateral bundle (ALB) and posteromedial bundle (PMB) grafts in the femur, the distal ends of the grafts are passed out the lateral portal. A smoothing tool (Sm) is used to prepare the tibial tunnel for graft passage and is passed from the anterior tibial aperture up and out the lateral portal. There, the distal passing sutures of the ALB and PMB grafts are passed through a loop (white arrow) in the Sm. The Sm is then used to pull the distal ends of the grafts back into the joint and down the tibial tunnel. The patient in this image has had a central third bone-patellar tendon-bone autograft taken for simultaneous anterior cruciate ligament reconstruction.
The PCL graft is then secured on the tibia. The ALB should be secured first at 90°, in neutral rotation, and with an anterior drawer to restore the tibiofemoral step-off. The graft is secured using a fully threaded, bicortical 6.5 mm cancellous screw, and an 18 mm spiked washer.[27] Alternatively, bone staples can also be used but usually cause more postoperative pain than screws and washers. The PMB should then be secured with the knee in full extension, utilizing the same-sized screw and washer with an anterior force applied to the tibia and distal traction on the grafts. On completion, a posterior drawer test with the knee flexed at 90° should be performed to confirm posterior stability.[27] Excess graft should be excised, and the wound closed with subcuticular sutures.
REHABILITATION
Postoperatively, patients should be non-weight bearing for a minimum of 6 weeks. They should transition from an immobilizer immediately after surgery to a dynamic PCL brace on adequate reduction of swelling, ideally in the first 4–5 days[50,85] [Figure 14]. The dynamic PCL brace should be worn at all times except for hygiene. Physical therapy should include initiation of prone passive knee flexion on post-operative day 1, with a limit of 90° of flexion in the first 2 weeks. Hyperextension is avoided to minimize strain on the healing PCL grafts. Early patellar mobilizations and passive motion, with the goal of reaching 90° of flexion within the first 2 weeks, are important to prevent arthrofibrosis. In addition, rehabilitation should focus on preventing PTT through either body positioning or muscle pulling forces with exercise. Early therapy focuses on patient education, symptom management, joint mobility, and quadriceps activation exercises. Neuromuscular electrical stimulation and blood flow restriction therapy are useful for recovering quadriceps muscle strength and reducing muscular atrophy during the most restrictive early rehabilitation period.[86,87] After 6 weeks, patients should begin weaning from crutches to gradually return to full weight bearing (FWB) and may initiate weight bearing exercise once FWB is well tolerated. Resisted hamstring curling into knee flexion and squatting >70° is restricted for the first 4 months to avoid deleterious PTT.[88,89] The patient’s therapy routine should become incrementally more demanding and evolve in structure to recover fitness, stability, strength, and power. Adjustments should be made to honor any knee joint irritability, observed through pain and swelling. Repeat kneeling stress radiographs should be evaluated at 6 months post-operative. If there is <2 mm posterior translation in comparison to other knee, patients can discontinue the use of the PCL brace and begin an impact exercise program. Patients with >2 mm translation, BMI >35.0, or a revision PCLR should wear the PCL brace at night until 1 year postoperatively. Functional testing should be performed between 9 and 12 months postoperatively to determine return to full activities. A PCL dynamic brace should be worn for the 1st year of return to athletics. The literature has reported that objective graft stretching, as measured by PCL stress radiographs, does not occur with this PT protocol and this protocol has allowed for decreased knee stiffness, quicker return to knee motion, and improved level of function.[50]
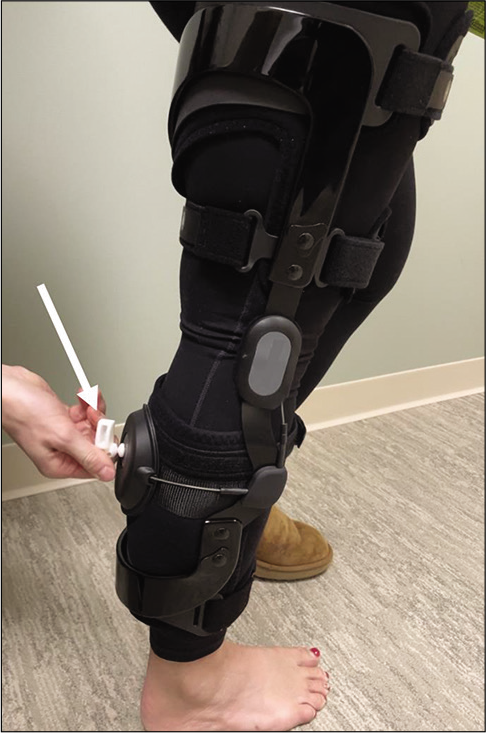
- Posterior cruciate ligament (PCL) dynamic brace: After reconstruction of the PCL, a dynamic support brace is recommended to prevent stretching of the graft. Graft stretching (or healing of the native ligament in an elongated position, as may happen with conservative management of PCL injuries) may lead to chronic instability and deficits in function. A dynamic brace provides anteriorly directed force below the knee and counteracts posteriorly directed forces on the lower limb. Some devices use a tensioning system, using a key (white arrow) to appropriately fit the brace to each patient. The device pictured above is an example dynamic PCL brace (Rebound PCL, Össur, Reykjavik, Iceland).
DISCUSSION
SB versus DB PCLR comparison
Anatomic DB-PCLR techniques have been reported to improve IKDC scores, reduce PTT, and restore the knee back to its native biomechanical function to a greater degree in comparison with the single bundle PCL reconstruction.[10,12,32,52]
A systematic review in 2017 compared SB-PCLR and DB-PCLR in 441 patients with a minimum of 2 years of follow-up.[10] Both SB and DB PCL procedures resulted in similar subjective outcomes, but IKDC scores were higher postoperatively in patients who had undergone double bundle in comparison to single bundle.[10] In addition, the authors reported that the objective difference in posterior laxity postoperatively between knees (as measured with a Telos device at 90°) was significantly reduced in patients undergoing a double bundle PCL reconstruction.[10]
Analyzing eight separate biomechanical studies, Lee et al. (2017) reported that SB PCLR resulted in a significantly greater PTT at all knee flexion angles, measured with a posterior drawer test, compared to DB PCLR.[12] Furthermore, a meta-analysis in 2015 concluded that surgeons may be better able to restore the knee back to native biomechanics utilizing a double bundle approach.[32]
Two prospective, randomized controlled trials reported higher IKDC scores and decreased posterior translation postoperatively after undergoing the double bundle procedure in comparison to the single bundle.[90,91] Yoon et al. analyzed 25 cases of SB reconstruction and 28 cases with DB reconstruction, finding that the DB approach was associated with significantly higher IKDC scores and showed less PTT in comparison to SB with minimum of 2-year follow-up.[90] Li et al. reported similar results; at a minimum of 2-year follow-up the DB cohort had a statistically higher IKDC scores of 71.6 in comparison to 65.5 in the SB cohort.[91] In addition, the DB group demonstrated significantly less tibial translation in comparison to the SB group.[91]
DB-PCL reconstruction outcomes
DB-PCLR has been shown to reduce posterior laxity and improve subjective patient outcomes at follow-up. [10,32,52,64,90,91] LaPrade et al. (2018) reported significantly improved PTT on stress radiographs following DB reconstruction surgery (pre-operative was 11.0 ± 3.5 mm and post-operative was 1.6 ± 2.0 mm) in 100 consecutive patients with a mean follow-up of 3 years. Significantly, these results from anatomic PCL reconstruction were comparable to outcomes of an isolated ACL reconstruction control cohort.[52] Other studies objectively measured PTT with stress radiographs and reported similar results in reduction of posterior laxity and have reported statistical improvement in subjective outcome scores including Tegner, Lysholm, Cincinnati, WOMAC, IKDC, and SF-12 PCS following DB reconstruction.[32,52,64,90,91] LaPrade et al. (2018) observed that the Tegner activity score improved from 2 to 5, the Lysholm score from 48.0 to 86.0, WOMAC score decreased from 35.5 to 5.0, and SF-12 PCS improved from 34.0 to 54.8 after a median 3-year follow-up.[52] Subjective scores should be utilized in combination with objective measurements such as PTT on stress radiographs and imaging modalities including MRI when assessing the success of the surgery at follow-up.
CONCLUSION
The PCL is the largest and strongest ligament of the knee.[22] Isolated tears to the PCL are relatively uncommon because the bulk of cases occur concurrently with other knee injuries, especially the PLC.[5,32,49,51,54] When evaluating isolated or combined PCL injuries, kneeling stress radiographs are a validated tool that should be used in objectively confirming the diagnosis. Much progress has been made in the treatment of both isolated and combined PCL tears, in terms of anatomic reconstruction techniques and improvements in both objective and subjective outcome measurements. Non-operative management with a dynamic knee brace can be utilized when the tear results in PTT <8 mm due to the PCL’s intrinsic healing ability, as it obtains its blood supply through the adjacent posterior capsule.[32,65] However, if the PTT is >8 mm or a multi-ligament injury occurred, ligament reconstruction should be performed to decrease the risk of osteoarthritis and to restore native knee mechanics.[32,50,53] Recent systematic reviews, meta-analyses, and the results from the work of many authors report the double-bundle approach is more effective in increasing IKDC scores, decreasing PTT, and better restoring the native biomechanics of the knee in comparison to the single-bundle approach.
Declaration of patient consent
Patient’s consent not required as patients identity is not disclosed or compromised.
Financial support and sponsorship
Nil.
Conflicts of interest
Consultant for Arthrex, Ossur, Smith and Nephew and Linvatec Royalties: Arthrex, Ossur and Smith and Nephew Research grants; Smith and Nephew and Ossur Editorial Boards: American Orthopaedic Society for Sports Medicine (AOSSM), Journal of Experimental Orthopaedics (JEO) and Knee Surgery, Sports Traumatology, Arthroscopy (KSSTA) Committees: AOSSM, Arthroscopic Association of North America (AANA), International Society of Arthroscopy, Knee Surgery, and Orthopaedic Sports Medicine (ISAKOS).
References
- The effect of posterior cruciate ligament deficiency on knee kinematics. Am J Sports Med. 2004;32:1915-22.
- [CrossRef] [PubMed] [Google Scholar]
- Diagnostic and incidence of the rupture of the posterior cruciate ligament. Unfallchirurg. 1999;102:753-62.
- [CrossRef] [PubMed] [Google Scholar]
- Interrater reliability in assessing quality of diagnostic accuracy studies using the QUADAS tool. A preliminary assessment. Acad Radiol. 2006;13:803-10.
- [CrossRef] [PubMed] [Google Scholar]
- Posterior cruciate ligament injuries in trauma patients. Arthroscopy. 1993;9:291-4.
- [CrossRef] [Google Scholar]
- Posterior cruciate ligament injuries in trauma patients: Part II. Arthrosc J Arthrosc Relat Surg. 1995;11:526-9.
- [CrossRef] [Google Scholar]
- Evaluation and treatment of posterior cruciate ligament injuries. Am J Sports Med. 1998;26:471-82.
- [CrossRef] [PubMed] [Google Scholar]
- Rupture of the posterior cruciate ligament: Status of current treatment. Unfallchirurg. 1996;99:382-99.
- [Google Scholar]
- The natural history of acute, isolated, nonoperatively treated posterior cruciate ligament injuries. A prospective study. Am J Sports Med. 1999;27:276-83.
- [CrossRef] [PubMed] [Google Scholar]
- Physical examination tests for the diagnosis of posterior cruciate ligament rupture: A systematic review. J Orthop Sports Phys Ther. 2013;43:804-13.
- [CrossRef] [PubMed] [Google Scholar]
- Single-bundle and double-bundle posterior cruciate ligament reconstructions: A systematic review and meta-analysis of 441 patients at a minimum 2 years' follow-up. Arthrosc J Arthrosc Relat Surg. 2017;33:2066-80.
- [CrossRef] [PubMed] [Google Scholar]
- Tibial slope strongly influences knee stability after posterior cruciate ligament reconstruction. Am J Sports Med. 2017;45:355-61.
- [CrossRef] [PubMed] [Google Scholar]
- Biomechanical comparison of single bundle and double-bundle posterior cruciate ligament reconstruction: A systematic review and meta-analysis. JBJS Rev. 2017;5:1-10.
- [CrossRef] [PubMed] [Google Scholar]
- Anatomy and biomechanics of the posterior cruciate ligament, medial and lateral sides of the knee. Sports Med Arthrosc. 2010;18:222-9.
- [CrossRef] [PubMed] [Google Scholar]
- Posterior cruciate ligament: Current concepts review. Arch bone Joint Surg. 2018;6:8-18.
- [Google Scholar]
- Long-term results of nonoperative treatment of isolated posterior cruciate ligament injuries in the athlete. Am J Sports Med. 1986;14:35-8.
- [CrossRef] [PubMed] [Google Scholar]
- Natural history of the posterior cruciate ligament-deficient knee. Clin Orthop Relat Res. 1989;246:208-16.
- [CrossRef] [Google Scholar]
- Arthroscopic evaluation of articular cartilage lesions in posterior cruciate ligament deficient knees. Arthrosc J Arthrosc Relat Surg. 2003;19:262-8.
- [CrossRef] [PubMed] [Google Scholar]
- Analysis of tibiofemoral cartilage deformation in the posterior cruciate ligament-deficient knee. J Bone Joint Surg Ser A. 2009;91:167-75.
- [CrossRef] [PubMed] [Google Scholar]
- Diagnosis and treatment of posterior cruciate ligament injuries. Curr Sports Med Rep. 2007;6:293-9.
- [CrossRef] [Google Scholar]
- Biomechanics of the PCL and related structures: Posterolateral, posteromedial and meniscofemoral ligaments. Knee Surg Sports Traumatol Arthrosc. 2003;11:271-81.
- [CrossRef] [PubMed] [Google Scholar]
- Lateral, posterior, and cruciate knee anatomy In: Noyes' Knee Disorders: Surgery. Rehabilitation, Clinical Outcomes. United States: Saunders; 2009. p. :20-43.
- [CrossRef] [PubMed] [Google Scholar]
- Arthroscopically pertinent anatomy of the anterolateral and posteromedial bundles of the posterior cruciate ligament. J Bone Joint Surg Ser A. 2012;94:1936-45.
- [CrossRef] [PubMed] [Google Scholar]
- The attachments of the fiber bundles of the posterior cruciate ligament: An anatomic study. Arthrosc J Arthrosc Relat Surg. 2007;23:284-90.
- [CrossRef] [PubMed] [Google Scholar]
- The cruciate ligaments of the knee joint. Anatomical functional and experimental analysis. Clin Orthop Relat Res. 1975;106:216-31.
- [CrossRef] [PubMed] [Google Scholar]
- Tibial insertion of the posterior cruciate ligament: A sagittal plane analysis using gross, histologic, and radiographic methods. Arthrosc J Arthrosc Relat Surg. 2008;24:269-75.
- [CrossRef] [PubMed] [Google Scholar]
- Quantitative analysis of human cruciate ligament insertions. Arthroscopy. 1999;15:741-9.
- [CrossRef] [Google Scholar]
- Posterior cruciate ligament graft fixation angles, part 1: Biomechanical evaluation for anatomic single-bundle reconstruction. Am J Sports Med. 2014;42:2338-45.
- [CrossRef] [PubMed] [Google Scholar]
- Topography of the femoral attachment of the posterior cruciate ligament. J Bone Joint Surg Ser A. 2008;90:249-55.
- [CrossRef] [PubMed] [Google Scholar]
- Anatomical study of the femoral and tibial insertions of the anterolateral and posteromedial bundles of human posterior cruciate ligament. Knee Surg Sport Traumatol Arthrosc. 2006;14:1055-9.
- [CrossRef] [PubMed] [Google Scholar]
- Codominance of the individual posterior cruciate ligament bundles: An analysis of bundle lengths and orientation. Am J Sports Med. 2003;31:221-5.
- [CrossRef] [PubMed] [Google Scholar]
- Kinematic analysis of the posterior cruciate ligament, part 1: The individual and collective function of the anterolateral and posteromedial bundles. Am J Sports Med. 2013;41:2828-38.
- [CrossRef] [PubMed] [Google Scholar]
- Emerging updates on the posterior cruciate ligament. Am J Sports Med. 2015;43:3077-92.
- [CrossRef] [PubMed] [Google Scholar]
- Dynamic three-dimensional computed tomography mapping of isometric posterior cruciate ligament attachment sites on the tibia and femur: Single versus double-bundle analysis. Arthrosc J Arthrosc Relat Surg. 2020;36:2875-84.
- [CrossRef] [PubMed] [Google Scholar]
- Topography of the femoral attachment of the posterior cruciate ligament: Surgical technique. J Bone Joint Surg Ser A. 2009;91(Suppl 2):89-100.
- [CrossRef] [PubMed] [Google Scholar]
- Surgical treatment of posterior cruciate ligament tears: An evolving technique. J Am Acad Orthop Surg. 2009;17:435-46.
- [CrossRef] [PubMed] [Google Scholar]
- Morphometric side-to-side differences in human cruciate ligament insertions. Surg Radiol Anat. 2006;28:398-402.
- [CrossRef] [PubMed] [Google Scholar]
- Posterior cruciate ligament: Anatomy and biomechanics. Curr Rev Musculoskelet Med. 2018;11:510-4.
- [CrossRef] [PubMed] [Google Scholar]
- Radiographic and anatomic landmarks of the major knee ligaments. J Bone Joint Surg Am. 2018;100:1241-50.
- [CrossRef] [PubMed] [Google Scholar]
- Radiographic landmarks for tunnel positioning in posterior cruciate ligament reconstructions. Am J Sports Med. 2013;41:35-42.
- [CrossRef] [PubMed] [Google Scholar]
- Posterior cruciate ligament: Anatomy, biomechanics, and outcomes. Am J Sports Med. 2012;40:222-31.
- [CrossRef] [PubMed] [Google Scholar]
- Anatomy of the posterior cruciate ligament and the meniscofemoral ligaments. Knee Surg Sport Traumatol Arthrosc. 2006;14:257-63.
- [CrossRef] [PubMed] [Google Scholar]
- Meniscofemoral ligaments revisited. J Bone Joint Surg Ser B. 2002;84:846-51.
- [CrossRef] [Google Scholar]
- Tension studies of human knee ligaments. Yield point ultimate failure, and disruption of the cruciate and tibial collateral ligaments. J Bone Joint Surg Ser A. 1976;58:350-5.
- [CrossRef] [Google Scholar]
- The mechanical properties of the two bundles of the human posterior cruciate ligament. J Biomech. 1994;27:13-24.
- [CrossRef] [Google Scholar]
- Long-term outcomes following single-bundle transtibial arthroscopic posterior cruciate ligament reconstruction. Int Orthop. 2013;37:337-43.
- [CrossRef] [PubMed] [Google Scholar]
- Posterior cruciate ligament reconstruction current trends. J Knee Surg. 2004;17:133-9.
- [CrossRef] [PubMed] [Google Scholar]
- The human posterior cruciate ligament complex: An interdisciplinary study: Ligament morphology and biomechanical evaluation. Am J Sports Med. 1995;23:736-45.
- [CrossRef] [PubMed] [Google Scholar]
- Ligamentous restraints to anterior-posterior drawer in the human knee. A biomechanical study. J Bone Joint Surg Am. 1980;62:259-70.
- [CrossRef] [PubMed] [Google Scholar]
- Stress radiography to measure posterior cruciate ligament insufficiency: A comparison of five different techniques. Knee Surg Sport Traumatol Arthrosc. 2006;14:1116-21.
- [CrossRef] [PubMed] [Google Scholar]
- Anatomic double-bundle posterior cruciate ligament reconstruction. Arthrosc Tech. 2016;5:e149-56.
- [CrossRef] [PubMed] [Google Scholar]
- Double-bundle posterior cruciate ligament reconstruction in 100 patients at a mean 3 years' follow-up: Outcomes were comparable to anterior cruciate ligament reconstructions. Am J Sports Med. 2018;46:1809-18.
- [CrossRef] [PubMed] [Google Scholar]
- Posterior cruciate ligament injures: A practical guide to management. J Bone Joint Surg. 2002;84:777.
- [CrossRef] [Google Scholar]
- Anatomy and biomechanics of the posterolateral corner of the knee. J Knee Surg. 2005;18:137-45.
- [CrossRef] [PubMed] [Google Scholar]
- PCL Tear: Complete, Partial, and Associated with Medial or Lateral Damage. 2016:307-23.
- [CrossRef] [Google Scholar]
- Correlation of valgus stress radiographs with medial knee ligament injuries: An in vitro biomechanical study. Am J Sports Med. 2010;38:330-8.
- [CrossRef] [PubMed] [Google Scholar]
- Accuracy of diagnoses from magnetic resonance imaging of the knee: A multi-center analysis of one thousand and fourteen patients. J Bone Joint Surg Ser A. 1991;73:2-10.
- [CrossRef] [Google Scholar]
- The accuracy of selective magnetic resonance imaging compared with the findings of arthroscopy of the knee. J Bone Joint Surg Ser A. 1988;70:192-8.
- [CrossRef] [Google Scholar]
- Analysis of low-field magnetic resonance imaging scanners for evaluation of knee pathology based on arthroscopy. Orthop J Sport Med. 2013;1:1-7.
- [CrossRef] [PubMed] [Google Scholar]
- Chronically injured posterior cruciate ligament: Magnetic resonance imaging. Clin Orthop Relat Res. 1997;335:224-32.
- [CrossRef] [Google Scholar]
- Stress radiography for the diagnosis of knee ligament injuries: A systematic review. Clin Orthop Relat Res. 2014;472:2644-57.
- [CrossRef] [PubMed] [Google Scholar]
- Intraobserver and interobserver reliability of the kneeling technique of stress radiography for the evaluation of posterior knee laxity. Am J Sports Med. 2008;36:1571-6.
- [CrossRef] [PubMed] [Google Scholar]
- Reliability of stress radiography for evaluation of posterior knee laxity. Am J Sports Med. 2005;33:502-6.
- [CrossRef] [PubMed] [Google Scholar]
- Isolated and combined grade-III posterior cruciate ligament tears treated with double-bundle reconstruction with use of endoscopically placed femoral tunnels and grafts: Operative technique and clinical outcomes. J Bone Joint Surg Ser A. 2011;93:1773-80.
- [CrossRef] [PubMed] [Google Scholar]
- Normal anatomy and biomechanics of the knee. Sports Med Arthrosc. 2011;19:82-92.
- [CrossRef] [PubMed] [Google Scholar]
- Ligaments and capsular structures In: Ligaments of the Knee. London, United Kingdom: Churchill Livingstone; 1995. p. :15-27.
- [Google Scholar]
- Posterior cruciate ligament tears: Functional and postoperative rehabilitation. Knee Surg Sports Traumatol Arthrosc. 2013;21:1071-84.
- [CrossRef] [PubMed] [Google Scholar]
- Nonoperatively treated isolated posterior cruciate ligament injuries. Am J Sports Med. 1993;21:132-6.
- [CrossRef] [PubMed] [Google Scholar]
- Lower limb biomechanics during level walking after an isolated posterior cruciate ligament rupture. Orthop J Sport Med. 2019;7:2325967119891164.
- [CrossRef] [PubMed] [Google Scholar]
- Long-term results of isolated anterolateral bundle reconstructions of the posterior cruciate ligament: A 6-to 12-year follow-up study. Am J Sports Med. 2009;37:1499-507.
- [CrossRef] [PubMed] [Google Scholar]
- Kinematic analysis of the posterior cruciate ligament, Part 2: A comparison of anatomic single versus double-bundle reconstruction. Am J Sports Med. 2013;41:2839-48.
- [CrossRef] [PubMed] [Google Scholar]
- Anterolateral transtibial posterior cruciate ligament reconstruction combined with anatomical reconstruction of posterolateral corner insufficiency: Comparison of single-bundle versus double-bundle posterior cruciate ligament reconstruction over a 2-to 6. Am J Sports Med. 2011;39:481-9.
- [CrossRef] [PubMed] [Google Scholar]
- Where should the femoral tunnel of a posterior cruciate ligament reconstruction be placed to best restore anteroposterior laxity and ligament forces? Am J Sports Med. 2006;34:604-11.
- [CrossRef] [PubMed] [Google Scholar]
- Function of posterior cruciate ligament bundles during in vivo knee flexion. Am J Sports Med. 2007;35:1507-12.
- [CrossRef] [PubMed] [Google Scholar]
- The role of osteotomy for the treatment of PCL injuries. Curr Rev Musculoskelet Med. 2018;11:298-306.
- [CrossRef] [PubMed] [Google Scholar]
- Role of osteotomy in multiligament knee injuries. Ann Joint. 2019;4:1-7.
- [CrossRef] [Google Scholar]
- Consequences of tibial tunnel reaming on the meniscal roots during cruciate ligament reconstruction in a cadaveric model, part 2: The posterior cruciate ligament. Am J Sports Med. 2015;43:200-6.
- [CrossRef] [PubMed] [Google Scholar]
- Biomechanical consequences of a complete radial tear adjacent to the medial meniscus posterior root attachment site: In situ pull-out repair restores derangement of joint mechanics. Am J Sports Med. 2014;42:699-707.
- [CrossRef] [PubMed] [Google Scholar]
- Biomechanical consequences of a tear of the posterior root of the medial meniscus: Similar to total meniscectomy. J Bone Joint Surg Ser A. 2008;90:1922-31.
- [CrossRef] [PubMed] [Google Scholar]
- Altered tibiofemoral contact mechanics due to lateral meniscus posterior horn root avulsions and radial tears can be restored with in situ pull-out suture repairs. J Bone Joint Surg Ser A. 2014;96:471-9.
- [CrossRef] [PubMed] [Google Scholar]
- Complications of posterior cruciate ligament surgery. Sports Med Arthrosc Rev. 2010;18:269-74.
- [CrossRef] [PubMed] [Google Scholar]
- How to avoid tunnel convergence in a multiligament injured knee. Ann Joint. 2018;3:1-5.
- [CrossRef] [Google Scholar]
- Multiple ligament reconstruction femoral tunnels: Intertunnel relationships and guidelines to avoid convergence. Am J Sports Med. 2017;45:563-9.
- [CrossRef] [PubMed] [Google Scholar]
- Intertunnel relationships in the tibia during reconstruction of multiple knee ligaments. Am J Sports Med. 2016;44:2864-9.
- [CrossRef] [PubMed] [Google Scholar]
- Quantification of functional brace forces for posterior cruciate ligament injuries on the knee joint: An in vivo investigation. Knee Surg Sport Traumatol Arthrosc. 2015;44:2864-9.
- [CrossRef] [PubMed] [Google Scholar]
- Quadriceps activation following knee injuries: A systematic review. J Athl Train. 2010;45:87-97.
- [CrossRef] [PubMed] [Google Scholar]
- Blood flow restriction training in clinical musculoskeletal rehabilitation: A systematic review and meta-analysis. Br J Sports Med. 2017;51:1003-11.
- [CrossRef] [PubMed] [Google Scholar]
- Effects of applied quadriceps and hamstrings muscle loads on forces in the anterior and posterior cruciate ligaments. Am J Sports Med. 2004;32:1144-9.
- [CrossRef] [PubMed] [Google Scholar]
- Cruciate ligament loading during common knee rehabilitation exercises. Proc Inst Mech Eng H. 2012;226:670-80.
- [CrossRef] [PubMed] [Google Scholar]
- A prospective randomized study comparing arthroscopic single-bundle and double-bundle posterior cruciate ligament reconstructions preserving remnant fibers. Am J Sports Med. 2011;39:474-80.
- [CrossRef] [PubMed] [Google Scholar]
- Comparison of single-bundle and double-bundle isolated posterior cruciate ligament reconstruction with allograft: A prospective, randomized study. Arthrosc J Arthrosc Relat Surg. 2014;30:695-700.
- [CrossRef] [PubMed] [Google Scholar]